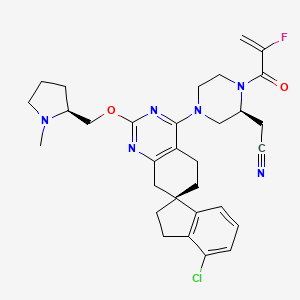
KRAS G12C inhibitor 44
- Click on QUICK INQUIRY to receive a quote from our team of experts.
- With the quality product at a COMPETITIVE price, you can focus more on your research.
Overview
Description
KRAS G12C inhibitor 44 is a small molecule that specifically targets the KRAS G12C mutation, a common genetic alteration found in various cancers, including non-small cell lung cancer, colorectal cancer, and pancreatic ductal adenocarcinoma . This compound has shown promise in inhibiting the KRAS G12C protein, which plays a crucial role in cancer cell proliferation and survival .
Preparation Methods
Synthetic Routes and Reaction Conditions: The synthetic route typically starts with a Boc-protected tetrahydropyridopyrimidine, followed by a regioselective nucleophilic aromatic substitution reaction to introduce a masked piperazine moiety . The final steps involve the installation of the prolinol subunit and the oxidation of the sulfide intermediate .
Industrial Production Methods: Industrial production of KRAS G12C inhibitor 44 involves scaling up the laboratory synthesis to kilogram-scale production. This process requires optimization of reaction conditions to ensure high yield and purity . The use of transition-metal-free oxidation and chromatography-free synthesis methods has significantly improved the efficiency of the industrial production process .
Chemical Reactions Analysis
Types of Reactions: KRAS G12C inhibitor 44 undergoes various chemical reactions, including nucleophilic aromatic substitution, oxidation, and palladium-catalyzed carbon-oxygen bond formation . These reactions are essential for the synthesis and modification of the compound.
Common Reagents and Conditions: Common reagents used in the synthesis of this compound include Boc-protected tetrahydropyridopyrimidine, prolinol, and masked piperazine . Reaction conditions often involve the use of organic solvents, controlled temperatures, and specific catalysts to achieve the desired transformations .
Major Products Formed: The major products formed during the synthesis of this compound include intermediates such as the sulfide intermediate and the final tetrahydropyridopyrimidine core with attached chiral building blocks .
Scientific Research Applications
KRAS G12C inhibitor 44 has numerous scientific research applications, particularly in the fields of chemistry, biology, medicine, and industry. In chemistry, it serves as a valuable tool for studying the reactivity and binding properties of KRAS G12C inhibitors . In biology, it is used to investigate the cellular and molecular mechanisms of KRAS G12C-driven cancers . In medicine, this compound is being evaluated in clinical trials for its potential to treat various cancers with KRAS G12C mutations . Additionally, it has industrial applications in the development of new cancer therapies and drug discovery .
Mechanism of Action
KRAS G12C inhibitor 44 exerts its effects by covalently binding to the KRAS G12C protein, locking it in an inactive state . This prevents the protein from transmitting pro-tumorigenic signals, thereby inhibiting cancer cell proliferation and survival . The compound specifically targets the cysteine residue at position 12 of the KRAS protein, which is mutated to glycine in KRAS G12C . This selective binding disrupts the guanine exchange cycle, effectively blocking the activation of downstream signaling pathways such as the RAF-MEK-ERK pathway .
Comparison with Similar Compounds
KRAS G12C inhibitor 44 is unique in its high selectivity and potency against the KRAS G12C mutation . Similar compounds include sotorasib and adagrasib, which also target the KRAS G12C protein but may differ in their pharmacokinetic properties and clinical efficacy . Another similar compound is 143D, which has shown comparable antitumor activity in preclinical models . These compounds share a common mechanism of action but may vary in their chemical structures and binding affinities .
List of Similar Compounds:- Sotorasib
- Adagrasib
- 143D
This compound stands out due to its optimized synthesis route and potential for high efficacy in treating KRAS G12C-driven cancers .
Properties
Molecular Formula |
C31H36ClFN6O2 |
---|---|
Molecular Weight |
579.1 g/mol |
IUPAC Name |
2-[(2S)-4-[(3R)-7-chloro-2'-[[(2S)-1-methylpyrrolidin-2-yl]methoxy]spiro[1,2-dihydroindene-3,7'-6,8-dihydro-5H-quinazoline]-4'-yl]-1-(2-fluoroprop-2-enoyl)piperazin-2-yl]acetonitrile |
InChI |
InChI=1S/C31H36ClFN6O2/c1-20(33)29(40)39-16-15-38(18-21(39)10-13-34)28-24-9-12-31(11-8-23-25(31)6-3-7-26(23)32)17-27(24)35-30(36-28)41-19-22-5-4-14-37(22)2/h3,6-7,21-22H,1,4-5,8-12,14-19H2,2H3/t21-,22-,31-/m0/s1 |
InChI Key |
LTBOVTGFAXPDLV-CYYCZYJVSA-N |
Isomeric SMILES |
CN1CCC[C@H]1COC2=NC3=C(CC[C@]4(C3)CCC5=C4C=CC=C5Cl)C(=N2)N6CCN([C@H](C6)CC#N)C(=O)C(=C)F |
Canonical SMILES |
CN1CCCC1COC2=NC3=C(CCC4(C3)CCC5=C4C=CC=C5Cl)C(=N2)N6CCN(C(C6)CC#N)C(=O)C(=C)F |
Origin of Product |
United States |
Disclaimer and Information on In-Vitro Research Products
Please be aware that all articles and product information presented on BenchChem are intended solely for informational purposes. The products available for purchase on BenchChem are specifically designed for in-vitro studies, which are conducted outside of living organisms. In-vitro studies, derived from the Latin term "in glass," involve experiments performed in controlled laboratory settings using cells or tissues. It is important to note that these products are not categorized as medicines or drugs, and they have not received approval from the FDA for the prevention, treatment, or cure of any medical condition, ailment, or disease. We must emphasize that any form of bodily introduction of these products into humans or animals is strictly prohibited by law. It is essential to adhere to these guidelines to ensure compliance with legal and ethical standards in research and experimentation.