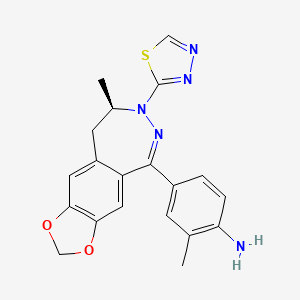
AMPA receptor antagonist-3
- Click on QUICK INQUIRY to receive a quote from our team of experts.
- With the quality product at a COMPETITIVE price, you can focus more on your research.
Overview
Description
AMPA receptor antagonist-3 is a compound that inhibits the activity of alpha-amino-3-hydroxy-5-methyl-4-isoxazolepropionic acid receptors. These receptors are a type of ionotropic glutamate receptor that mediate fast synaptic transmission in the central nervous system. AMPA receptor antagonists are of significant interest due to their potential therapeutic applications in neurological disorders such as epilepsy, depression, and neurodegenerative diseases.
Preparation Methods
Synthetic Routes and Reaction Conditions: The synthesis of AMPA receptor antagonist-3 typically involves the use of quinoxaline-2,3-dione derivatives. The synthetic route includes the following steps:
Formation of Quinoxaline Core: The reaction of o-phenylenediamine with glyoxal or its derivatives under acidic conditions to form the quinoxaline core.
Functionalization: Introduction of various functional groups at specific positions on the quinoxaline ring to enhance the antagonist activity. This step often involves reactions such as nitration, reduction, and alkylation.
Purification: The final product is purified using techniques such as recrystallization or chromatography to obtain a high-purity compound.
Industrial Production Methods: Industrial production of this compound follows similar synthetic routes but on a larger scale. The process involves:
Batch or Continuous Flow Reactors: To ensure consistent quality and yield.
Automated Purification Systems: For efficient separation and purification of the final product.
Quality Control: Rigorous testing to ensure the compound meets the required specifications.
Types of Reactions:
Oxidation: this compound can undergo oxidation reactions, often using reagents such as potassium permanganate or hydrogen peroxide.
Reduction: Reduction reactions can be performed using agents like sodium borohydride or lithium aluminum hydride.
Substitution: Various substitution reactions can be carried out to introduce different functional groups, using reagents like halogens or alkylating agents.
Common Reagents and Conditions:
Oxidation: Potassium permanganate in acidic or neutral conditions.
Reduction: Sodium borohydride in methanol or ethanol.
Substitution: Halogens (chlorine, bromine) in the presence of a catalyst.
Major Products:
Oxidation Products: Oxidized derivatives of the quinoxaline ring.
Reduction Products: Reduced forms of the functional groups on the quinoxaline ring.
Substitution Products: Various substituted quinoxaline derivatives with enhanced antagonist activity.
Scientific Research Applications
AMPA receptor antagonist-3 has a wide range of scientific research applications:
Chemistry: Used as a tool to study the structure-activity relationship of ionotropic glutamate receptors.
Biology: Helps in understanding the role of AMPA receptors in synaptic plasticity and memory formation.
Medicine: Potential therapeutic agent for treating neurological disorders such as epilepsy, depression, and neurodegenerative diseases.
Industry: Used in the development of new drugs targeting AMPA receptors.
Mechanism of Action
AMPA receptor antagonist-3 exerts its effects by binding to the ligand-binding domain of alpha-amino-3-hydroxy-5-methyl-4-isoxazolepropionic acid receptors, preventing the binding of glutamate. This inhibition blocks the ion flow through the receptor, reducing neuronal excitation. The molecular targets include the GluA1-GluA4 subunits of the receptor, and the pathways involved are primarily related to synaptic transmission and plasticity.
Comparison with Similar Compounds
Perampanel: A non-competitive AMPA receptor antagonist used in the treatment of epilepsy.
GluA2 Antagonists: Compounds that specifically target the GluA2 subunit of AMPA receptors.
Uniqueness: AMPA receptor antagonist-3 is unique due to its specific binding affinity and selectivity for the alpha-amino-3-hydroxy-5-methyl-4-isoxazolepropionic acid receptor subunits, making it a valuable tool for both research and therapeutic applications.
Properties
Molecular Formula |
C20H19N5O2S |
---|---|
Molecular Weight |
393.5 g/mol |
IUPAC Name |
2-methyl-4-[(8R)-8-methyl-7-(1,3,4-thiadiazol-2-yl)-8,9-dihydro-[1,3]dioxolo[4,5-h][2,3]benzodiazepin-5-yl]aniline |
InChI |
InChI=1S/C20H19N5O2S/c1-11-5-13(3-4-16(11)21)19-15-8-18-17(26-10-27-18)7-14(15)6-12(2)25(24-19)20-23-22-9-28-20/h3-5,7-9,12H,6,10,21H2,1-2H3/t12-/m1/s1 |
InChI Key |
HCOJNYUPUOBYDH-GFCCVEGCSA-N |
Isomeric SMILES |
C[C@@H]1CC2=CC3=C(C=C2C(=NN1C4=NN=CS4)C5=CC(=C(C=C5)N)C)OCO3 |
Canonical SMILES |
CC1CC2=CC3=C(C=C2C(=NN1C4=NN=CS4)C5=CC(=C(C=C5)N)C)OCO3 |
Origin of Product |
United States |
Disclaimer and Information on In-Vitro Research Products
Please be aware that all articles and product information presented on BenchChem are intended solely for informational purposes. The products available for purchase on BenchChem are specifically designed for in-vitro studies, which are conducted outside of living organisms. In-vitro studies, derived from the Latin term "in glass," involve experiments performed in controlled laboratory settings using cells or tissues. It is important to note that these products are not categorized as medicines or drugs, and they have not received approval from the FDA for the prevention, treatment, or cure of any medical condition, ailment, or disease. We must emphasize that any form of bodily introduction of these products into humans or animals is strictly prohibited by law. It is essential to adhere to these guidelines to ensure compliance with legal and ethical standards in research and experimentation.