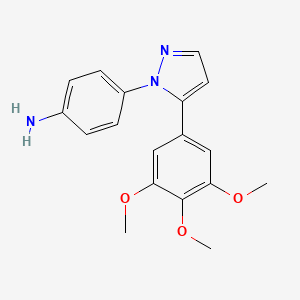
Tubulin inhibitor 32
- Click on QUICK INQUIRY to receive a quote from our team of experts.
- With the quality product at a COMPETITIVE price, you can focus more on your research.
Overview
Description
Tubulin inhibitor 32 (CAS: 2923531-39-7) is an orally active microtubule-targeting agent that inhibits tubulin polymerization, leading to cell cycle arrest at the G2/M phase and apoptosis in cancer cells . Preclinical studies highlight its anti-proliferative activity across various cancer models, though specific IC50 values remain undisclosed in the provided evidence. Its oral bioavailability and ability to disrupt microtubule networks position it as a promising candidate for cancer therapy, particularly in tumors dependent on microtubule dynamics for proliferation .
Preparation Methods
Synthetic Routes and Reaction Conditions
Tubulin inhibitor 32 is synthesized through a series of chemical reactions involving indole-acrylamide derivatives. The synthesis typically involves the following steps:
Formation of Indole Derivative: The indole derivative is synthesized through a Fischer indole synthesis reaction.
Acrylamide Formation: The acrylamide moiety is introduced through a reaction with acryloyl chloride in the presence of a base such as triethylamine.
Coupling Reaction: The indole derivative is then coupled with the acrylamide moiety using a coupling reagent such as N,N’-dicyclohexylcarbodiimide (DCC) to form the final product, this compound.
Industrial Production Methods
Industrial production of this compound involves scaling up the synthetic routes mentioned above. The process is optimized for large-scale production by adjusting reaction conditions, such as temperature, pressure, and solvent choice, to ensure high yield and purity. Advanced purification techniques, such as recrystallization and chromatography, are employed to obtain the final product .
Chemical Reactions Analysis
Types of Reactions
Tubulin inhibitor 32 undergoes various chemical reactions, including:
Oxidation: this compound can be oxidized using oxidizing agents such as potassium permanganate or hydrogen peroxide.
Reduction: Reduction reactions can be carried out using reducing agents like sodium borohydride or lithium aluminum hydride.
Common Reagents and Conditions
Oxidation: Potassium permanganate, hydrogen peroxide; acidic or basic conditions.
Reduction: Sodium borohydride, lithium aluminum hydride; solvent such as ethanol or tetrahydrofuran.
Substitution: Halogens (chlorine, bromine), alkylating agents (methyl iodide); solvent such as dichloromethane or acetonitrile.
Major Products Formed
Oxidation: Formation of oxidized derivatives with additional oxygen-containing functional groups.
Reduction: Formation of reduced derivatives with hydrogenated functional groups.
Substitution: Formation of substituted derivatives with new functional groups replacing the original ones.
Scientific Research Applications
Efficacy Against Cancer Cell Lines
Research has highlighted the efficacy of Tubulin inhibitor 32 against various cancer cell lines:
- Non-Small Cell Lung Cancer (A549) : this compound exhibited an IC50 value between 3–5 μM, effectively inducing cytotoxicity and leading to cell cycle arrest at the G2/M phase .
- HeLa and HL-60 : In these cell lines, this compound caused significant apoptosis and showed substantial inhibition of tubulin polymerization with IC50 values indicating high potency .
- Other Cancer Types : The compound has been tested against a range of other cancer types, demonstrating broad-spectrum anticancer activity. For instance, derivatives of this compound have been synthesized and evaluated for their antiproliferative effects against multiple cancer lines with varying degrees of success .
Study on Non-Small Cell Lung Cancer
In a detailed study involving non-small cell lung cancer cells, researchers treated A549 cells with this compound. The findings indicated:
- Cell Cycle Arrest : Cells were arrested at the G2/M phase within 12–18 hours post-treatment.
- Apoptotic Pathway Activation : Significant activation of apoptotic markers was observed, confirming that the compound induces cell death through programmed mechanisms .
Comparative Efficacy Study
A comparative study evaluated this compound against other known tubulin inhibitors. The results indicated:
Compound | IC50 (μM) | Mechanism | Cancer Cell Line Tested |
---|---|---|---|
This compound | 3–5 | Inhibition of tubulin polymerization | A549 |
Colchicine | 2.52 | Inhibition of tubulin polymerization | Various |
Paclitaxel | 0.1 | Promotion of tubulin polymerization | Various |
This table illustrates that while this compound is effective, other compounds like paclitaxel may exhibit superior potency in specific contexts.
Mechanism of Action
Tubulin inhibitor 32 exerts its effects by binding to the colchicine binding site on tubulin. This binding prevents tubulin from polymerizing into microtubules, leading to the disruption of the microtubule network. As a result, cells are unable to progress through the cell cycle, leading to cell cycle arrest at the G2/M phase and subsequent apoptosis. The compound also activates caspase-3, a key enzyme involved in the apoptotic pathway .
Comparison with Similar Compounds
Structural and Mechanistic Overview
Tubulin inhibitors targeting the colchicine-binding site share a common mechanism: destabilizing microtubules by preventing tubulin dimer polymerization. However, structural diversity leads to differences in potency, pharmacokinetics, and resistance profiles. Below is a comparative analysis of Tubulin inhibitor 32 and key analogs:
Key Compounds and Comparative Data
Critical Analysis
- Pyrrol-2(3H)-one derivatives exhibit stronger binding affinity (ΔG = -12.99 kcal/mol) than combretastatin A-4 (ΔG = -8.87 kcal/mol), suggesting enhanced target engagement .
- Oral Bioavailability: Both this compound and VERU-111 are orally available, a significant advantage over intravenous agents like paclitaxel .
- Resistance Mechanisms: VERU-111 uniquely overcomes Vemurafenib resistance in BRAF-mutant melanomas by downregulating Skp2-AKT signaling . In contrast, L1 targets βIII-tubulin-mediated resistance, a common evasion mechanism in taxane-resistant cancers .
- Synergy : VERU-111 synergizes with BRAF inhibitors, while L1 enhances efficacy with Plk1 inhibitors, highlighting divergent combinatorial strategies .
Clinical and Preclinical Advancements
- VERU-111: Currently in Phase 1b/2 trials for prostate cancer, with robust in vivo tumor growth inhibition (96.1% TGI in melanoma models) .
- This compound: Limited data on clinical progression, though its oral activity and apoptosis induction align with trends in next-generation tubulin inhibitors .
- ABI-274 : Served as a tool compound for structural optimization, leading to VERU-111’s development .
Q & A
Basic Research Questions
Q. How can researchers determine the IC50 of Tubulin Inhibitor 32 in vitro, and what methodological considerations are critical for reproducibility?
To measure IC50, use dose-response experiments with tubulin polymerization assays (e.g., ELISA) to quantify inhibition efficiency. Ensure consistency in buffer conditions (pH, temperature) and tubulin concentration. Normalize data against controls (e.g., colchicine as a reference inhibitor) and validate with triplicate runs to minimize variability .
Q. What experimental controls are essential when assessing this compound’s impact on apoptosis in cancer cell lines?
Include negative controls (untreated cells), positive controls (cells treated with established apoptosis inducers like staurosporine), and vehicle controls (e.g., DMSO used for compound solubilization). Use flow cytometry with Annexin V/PI staining to distinguish apoptotic vs. necrotic cells, and corroborate with caspase-3/7 activity assays .
Q. How should researchers address discrepancies in this compound’s reported efficacy across different cancer cell types?
Analyze cell line-specific factors such as tubulin isoform expression (e.g., βIII-tubulin overexpression in resistant cancers) and drug efflux pump activity (e.g., P-glycoprotein). Combine proliferation assays (MTT/XTT) with live-cell imaging to correlate cytotoxicity with mitotic arrest duration .
Advanced Research Questions
Q. What computational strategies can predict this compound’s binding affinity to tubulin’s colchicine site, and how can these be validated experimentally?
Perform molecular docking using tubulin’s crystal structure (PDB ID 1SA0) to model inhibitor interactions. Prioritize fragments with high binding energy at the colchicine pocket. Validate predictions with surface plasmon resonance (SPR) for kinetic analysis and competitive binding assays using fluorescent colchicine analogs (e.g., BODIPY-colchicine) .
Q. How can researchers design combination therapies to overcome resistance to this compound in aggressive tumors?
Screen synergistic partners using high-throughput combinatorial drug libraries. Focus on agents targeting resistance mechanisms (e.g., HDAC inhibitors to modulate βIII-tubulin expression or ABC transporter inhibitors to block efflux). Validate synergy via Chou-Talalay analysis (combination index <1 indicates synergy) .
Q. What structural modifications to this compound could enhance its blood-brain barrier (BBB) penetration for glioblastoma applications?
Apply Lipinski’s Rule of Five to optimize logP (<5) and polar surface area (<90 Ų). Introduce halogen atoms or methyl groups to improve lipophilicity. Test BBB permeability using in vitro models (e.g., hCMEC/D3 monolayers) and in vivo via PET imaging with radiolabeled analogs .
Q. How should researchers analyze conflicting data on this compound’s off-target effects in non-cancerous cells?
Use transcriptomics (RNA-seq) and proteomics (LC-MS/MS) to identify off-target pathways. Compare dose-response curves between cancerous and normal cells (e.g., fibroblasts) to establish therapeutic windows. Validate findings with CRISPR knockouts of suspected off-target genes .
Q. Methodological Frameworks
- For IC50 Validation : Combine tubulin polymerization assays with cell-based viability tests to confirm target-specific effects .
- For Combination Therapy Design : Use factorial experimental designs to test multiple drug ratios and dosing schedules .
- For Structural Optimization : Employ fragment-based drug design (FBDD) guided by tubulin’s binding pocket topology .
Properties
Molecular Formula |
C18H19N3O3 |
---|---|
Molecular Weight |
325.4 g/mol |
IUPAC Name |
4-[5-(3,4,5-trimethoxyphenyl)pyrazol-1-yl]aniline |
InChI |
InChI=1S/C18H19N3O3/c1-22-16-10-12(11-17(23-2)18(16)24-3)15-8-9-20-21(15)14-6-4-13(19)5-7-14/h4-11H,19H2,1-3H3 |
InChI Key |
WUKGECOHTOGGGA-UHFFFAOYSA-N |
Canonical SMILES |
COC1=CC(=CC(=C1OC)OC)C2=CC=NN2C3=CC=C(C=C3)N |
Origin of Product |
United States |
Disclaimer and Information on In-Vitro Research Products
Please be aware that all articles and product information presented on BenchChem are intended solely for informational purposes. The products available for purchase on BenchChem are specifically designed for in-vitro studies, which are conducted outside of living organisms. In-vitro studies, derived from the Latin term "in glass," involve experiments performed in controlled laboratory settings using cells or tissues. It is important to note that these products are not categorized as medicines or drugs, and they have not received approval from the FDA for the prevention, treatment, or cure of any medical condition, ailment, or disease. We must emphasize that any form of bodily introduction of these products into humans or animals is strictly prohibited by law. It is essential to adhere to these guidelines to ensure compliance with legal and ethical standards in research and experimentation.