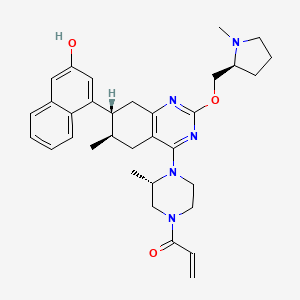
KRAS inhibitor-21
- Click on QUICK INQUIRY to receive a quote from our team of experts.
- With the quality product at a COMPETITIVE price, you can focus more on your research.
Overview
Description
KRAS inhibitor-21 is a small molecule compound designed to target and inhibit the activity of the Kirsten rat sarcoma viral oncogene homolog (KRAS) protein. KRAS is a small guanine triphosphatase (GTPase) that plays a pivotal role in cellular pathways governing cell survival, proliferation, and differentiation. Mutations in the KRAS gene are among the most common oncogenic drivers in human cancers, particularly in non-small cell lung cancer, colorectal cancer, and pancreatic cancer .
Preparation Methods
Synthetic Routes and Reaction Conditions
The synthesis of KRAS inhibitor-21 involves multiple steps, including the formation of key intermediates and the final coupling reactions. The synthetic route typically starts with the preparation of a core scaffold, followed by functional group modifications to introduce specific substituents that enhance the binding affinity and selectivity for the KRAS protein. Common reaction conditions include the use of organic solvents, catalysts, and controlled temperature and pressure settings .
Industrial Production Methods
Industrial production of this compound involves scaling up the laboratory synthesis to a larger scale while ensuring consistency, purity, and yield. This process often requires optimization of reaction conditions, purification techniques, and quality control measures. Techniques such as high-performance liquid chromatography (HPLC) and mass spectrometry are employed to monitor the purity and identity of the compound .
Chemical Reactions Analysis
Types of Reactions
KRAS inhibitor-21 undergoes various chemical reactions, including:
Oxidation: The compound can be oxidized under specific conditions to form oxidized derivatives.
Reduction: Reduction reactions can be used to modify certain functional groups within the molecule.
Substitution: Substitution reactions are employed to introduce or replace functional groups, enhancing the compound’s activity and selectivity.
Common Reagents and Conditions
Common reagents used in these reactions include oxidizing agents (e.g., hydrogen peroxide), reducing agents (e.g., sodium borohydride), and nucleophiles (e.g., amines). Reaction conditions such as temperature, solvent choice, and reaction time are carefully controlled to achieve the desired outcomes .
Major Products Formed
The major products formed from these reactions include various derivatives of this compound with modified functional groups. These derivatives are often tested for their biological activity and selectivity towards the KRAS protein .
Scientific Research Applications
Colorectal Cancer
Recent research indicates that KRAS inhibitor-21 may play a significant role in preventing metachronous metastasis in colorectal cancer. A study utilized machine learning to develop a risk scoring model for predicting metastasis in KRAS-mutant colorectal cancer patients. The findings revealed that treatment with KRAS inhibitors significantly suppressed migration and invasion capabilities of high-risk colorectal cancer cells by inhibiting epithelial-mesenchymal transition (EMT) and TGF-β signaling pathways .
Table 1: Effects of this compound on Colorectal Cancer Models
Model Type | Treatment | Migration Inhibition (%) | EMT Marker Changes |
---|---|---|---|
SW837 (KRAS G12C) | This compound | 70% | Decreased N-cadherin, increased E-cadherin |
PDO-CC0117 (KRAS G12D) | This compound | 65% | Decreased vimentin, increased E-cadherin |
Lung Cancer
In non-small cell lung cancer (NSCLC), the combination of this compound with other targeted therapies has shown promise. Clinical trials combining KRAS inhibitors with MEK inhibitors have demonstrated improved progression-free survival rates compared to monotherapy . The synergy between these agents is attributed to their complementary mechanisms in disrupting the RAS/MAPK pathway.
Table 2: Clinical Trial Outcomes for NSCLC
Trial Name | Combination Therapy | Objective Response Rate (%) | Median Progression-Free Survival (Months) |
---|---|---|---|
CodeBreak 100 | Sotorasib (KRAS G12C Inhibitor) | 37.1 | 6.8 |
KRYSTAL-1 | Adagrasib (KRAS G12C Inhibitor) | 42.9 | 6.5 |
Case Study 1: Treatment of KRAS-Mutant Adenocarcinoma
A notable case involved a patient with advanced KRAS-mutant adenocarcinoma treated with a combination of MEK inhibitors and docetaxel. The results indicated a significant improvement in progression-free survival from 2.1 months with docetaxel alone to 5.3 months when combined with MEK inhibitors . This case exemplifies the potential of combination therapies involving KRAS inhibitors.
Case Study 2: Resistance Mechanisms
Despite the efficacy of this compound, resistance mechanisms have been observed, particularly in colorectal cancer models where prolonged treatment led to increased IC50 values and maintained p-ERK levels . Understanding these resistance pathways is crucial for developing strategies to enhance the effectiveness of treatment regimens.
Mechanism of Action
KRAS inhibitor-21 exerts its effects by binding to the KRAS protein and inhibiting its activity. The compound specifically targets the guanine triphosphate (GTP)-bound active form of KRAS, preventing its interaction with downstream effectors such as RAF, MEK, and ERK. This inhibition disrupts the KRAS-mediated signaling pathways, leading to reduced cell proliferation and increased apoptosis in KRAS-mutant cancer cells .
Comparison with Similar Compounds
KRAS inhibitor-21 is unique compared to other KRAS inhibitors due to its specific binding affinity and selectivity for the KRAS protein. Similar compounds include:
Sotorasib (AMG 510): A selective KRAS G12C inhibitor with proven clinical efficacy.
Adagrasib (MRTX849): Another selective KRAS G12C inhibitor with similar mechanisms of action.
BI 2852: A pan-KRAS inhibitor that targets multiple KRAS isoforms.
This compound stands out due to its unique chemical structure and binding properties, which contribute to its potency and selectivity in targeting KRAS-mutant cancers .
Biological Activity
KRAS is a pivotal oncogene frequently mutated in various cancers, notably pancreatic ductal adenocarcinoma (PDAC), lung cancer, and colorectal cancer. The development of KRAS inhibitors, particularly KRAS inhibitor-21, represents a significant advancement in targeted cancer therapy. This article explores the biological activity of this compound, focusing on its mechanism of action, efficacy against different KRAS mutations, and associated resistance mechanisms.
This compound functions primarily by binding to the inactive GDP-bound state of KRAS, inhibiting nucleotide exchange and thereby blocking downstream signaling pathways critical for tumor growth and survival. Research indicates that the inhibitor exhibits a mean IC50 value of approximately 5 nM for inactive KRAS states, while its effect on active KRAS mutants requires higher concentrations (IC50 around 5.5 µM) . This differential binding affinity underscores the potential for selective targeting of mutant KRAS forms while sparing wild-type variants.
Efficacy Against KRAS Mutations
The efficacy of this compound has been evaluated across various models expressing common KRAS mutations. It has demonstrated the ability to inhibit 18 out of the 24 most prevalent KRAS mutations found in cancer . The following table summarizes the activity of this compound against specific KRAS mutations:
KRAS Mutation | IC50 (nM) | Response |
---|---|---|
G12C | 1 | High inhibition |
G12D | 2 | High inhibition |
G12V | 10 | Moderate inhibition |
G13D | 15 | Moderate inhibition |
Q61H | 20 | Lower inhibition |
Case Studies
Case Study 1: Pancreatic Ductal Adenocarcinoma (PDAC)
In preclinical models of PDAC harboring KRAS mutations, treatment with this compound resulted in significant tumor regression and prolonged survival compared to control groups. The study highlighted that while the inhibitor effectively targeted mutant KRAS, resistance mechanisms, such as upregulation of compensatory pathways (e.g., macropinocytosis), were observed .
Case Study 2: Non-Small Cell Lung Cancer (NSCLC)
A cohort study involving patients with NSCLC and G12C mutations treated with this compound showed an overall response rate (ORR) of 45%, with durable responses noted in several cases . Notably, co-existing mutations in STK11 were associated with varied responses, indicating the complexity of resistance mechanisms.
Resistance Mechanisms
Resistance to KRAS inhibitors like this compound can arise through several mechanisms:
- Mutational Escape : Secondary mutations in the KRAS gene can alter binding sites, reducing drug affinity.
- Pathway Compensation : Activation of alternative signaling pathways (e.g., RTKs) can bypass the inhibited pathway .
- Deubiquitinase Activity : Studies have identified USP21 as a potential mediator of resistance by promoting macropinocytosis and enhancing nutrient uptake in cells lacking active KRAS .
Properties
Molecular Formula |
C33H41N5O3 |
---|---|
Molecular Weight |
555.7 g/mol |
IUPAC Name |
1-[(3S)-4-[(6R,7R)-7-(3-hydroxynaphthalen-1-yl)-6-methyl-2-[[(2S)-1-methylpyrrolidin-2-yl]methoxy]-5,6,7,8-tetrahydroquinazolin-4-yl]-3-methylpiperazin-1-yl]prop-2-en-1-one |
InChI |
InChI=1S/C33H41N5O3/c1-5-31(40)37-13-14-38(22(3)19-37)32-29-15-21(2)27(28-17-25(39)16-23-9-6-7-11-26(23)28)18-30(29)34-33(35-32)41-20-24-10-8-12-36(24)4/h5-7,9,11,16-17,21-22,24,27,39H,1,8,10,12-15,18-20H2,2-4H3/t21-,22+,24+,27-/m1/s1 |
InChI Key |
QFHHSQNNGXFEMZ-SMAWTLEESA-N |
Isomeric SMILES |
C[C@@H]1CC2=C(C[C@H]1C3=CC(=CC4=CC=CC=C43)O)N=C(N=C2N5CCN(C[C@@H]5C)C(=O)C=C)OC[C@@H]6CCCN6C |
Canonical SMILES |
CC1CC2=C(CC1C3=CC(=CC4=CC=CC=C43)O)N=C(N=C2N5CCN(CC5C)C(=O)C=C)OCC6CCCN6C |
Origin of Product |
United States |
Disclaimer and Information on In-Vitro Research Products
Please be aware that all articles and product information presented on BenchChem are intended solely for informational purposes. The products available for purchase on BenchChem are specifically designed for in-vitro studies, which are conducted outside of living organisms. In-vitro studies, derived from the Latin term "in glass," involve experiments performed in controlled laboratory settings using cells or tissues. It is important to note that these products are not categorized as medicines or drugs, and they have not received approval from the FDA for the prevention, treatment, or cure of any medical condition, ailment, or disease. We must emphasize that any form of bodily introduction of these products into humans or animals is strictly prohibited by law. It is essential to adhere to these guidelines to ensure compliance with legal and ethical standards in research and experimentation.