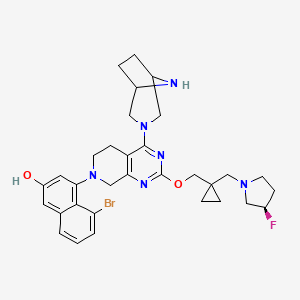
KRAS G12D inhibitor 8
- Click on QUICK INQUIRY to receive a quote from our team of experts.
- With the quality product at a COMPETITIVE price, you can focus more on your research.
Overview
Description
KRAS G12D inhibitor 8 is a small molecule designed to target the KRAS G12D mutation, which is a prevalent oncogenic driver in various cancers, particularly pancreatic ductal adenocarcinoma. This mutation results in the substitution of glycine with aspartic acid at position 12 of the KRAS protein, leading to continuous activation of downstream signaling pathways that promote cancer cell proliferation and survival .
Preparation Methods
Synthetic Routes and Reaction Conditions
The synthesis of KRAS G12D inhibitor 8 involves multiple steps, including the formation of key intermediates and final coupling reactions. One common approach is the use of structure-based virtual screening and molecular dynamics simulations to identify potential lead compounds . The synthetic route typically involves:
Formation of the core scaffold: This step includes the construction of the central molecular framework, often through cyclization reactions.
Functionalization: Introduction of functional groups that enhance binding affinity and specificity to the KRAS G12D protein.
Final coupling: The final step involves coupling the functionalized intermediates to form the complete inhibitor molecule.
Industrial Production Methods
Industrial production of this compound requires optimization of the synthetic route to ensure high yield and purity. This involves scaling up the reactions, optimizing reaction conditions (e.g., temperature, solvent, catalysts), and implementing purification techniques such as crystallization and chromatography .
Chemical Reactions Analysis
Types of Reactions
KRAS G12D inhibitor 8 undergoes various chemical reactions, including:
Oxidation: Introduction of oxygen atoms to form oxides or hydroxides.
Reduction: Removal of oxygen atoms or addition of hydrogen atoms.
Substitution: Replacement of one functional group with another.
Common Reagents and Conditions
Oxidation: Common oxidizing agents include potassium permanganate and hydrogen peroxide.
Reduction: Reducing agents such as sodium borohydride and lithium aluminum hydride are used.
Substitution: Reagents like halogens (chlorine, bromine) and nucleophiles (amines, thiols) are employed.
Major Products Formed
The major products formed from these reactions depend on the specific functional groups involved. For example, oxidation may yield ketones or carboxylic acids, while reduction can produce alcohols or amines .
Scientific Research Applications
KRAS G12D inhibitor 8 has significant applications in scientific research, particularly in the fields of:
Chemistry: Used as a tool compound to study the chemical properties and reactivity of KRAS G12D mutations.
Biology: Employed in cellular and molecular biology to investigate the role of KRAS G12D in cell signaling and cancer progression.
Industry: Utilized in the development of targeted cancer therapies and precision medicine approaches.
Mechanism of Action
KRAS G12D inhibitor 8 exerts its effects by binding to the KRAS G12D protein and preventing its interaction with downstream effectors such as RAF1. This inhibition disrupts the nucleotide exchange process and blocks the activation of signaling pathways like MAPK and PI3K, ultimately leading to reduced cancer cell proliferation and survival .
Comparison with Similar Compounds
KRAS G12D inhibitor 8 is unique in its high specificity and potency against the KRAS G12D mutation. Similar compounds include:
These compounds share similar mechanisms of action but differ in their chemical structures and binding affinities, highlighting the uniqueness of this compound in targeting the KRAS G12D mutation .
Biological Activity
KRAS mutations, particularly the G12D variant, are prevalent in various cancers, notably pancreatic ductal adenocarcinoma (PDAC). The development of specific inhibitors targeting KRAS G12D has become a focal point in cancer research. This article explores the biological activity of KRAS G12D inhibitor 8, alongside relevant case studies and findings from recent research.
Overview of KRAS G12D and Its Implications in Cancer
The KRAS gene encodes a protein that plays a critical role in cell signaling pathways regulating cell growth and survival. The G12D mutation leads to constitutive activation of KRAS, promoting oncogenic signaling and contributing to tumorigenesis. This mutation is found in approximately 45% of KRAS mutations in pancreatic cancer, making it a significant target for therapeutic intervention .
This compound functions by binding to the mutant KRAS protein, disrupting its interaction with downstream effectors. This inhibition prevents the activation of critical signaling pathways such as MAPK and PI3K, which are essential for cancer cell proliferation and survival. The compound's specificity for the G12D variant allows for targeted therapy with potentially reduced off-target effects .
Efficacy Studies
Recent studies have demonstrated the efficacy of this compound in various preclinical models:
- In Vitro Studies : In cellular assays, inhibitor 8 exhibited sub-nanomolar binding affinity for KRAS G12D, leading to significant reductions in cell viability and proliferation in PDAC cell lines . The compound's potency was confirmed through dose-response experiments.
- In Vivo Studies : Animal models bearing KRAS G12D-driven tumors treated with inhibitor 8 showed marked tumor regression. For instance, treatment resulted in complete or near-complete remissions within two weeks, accompanied by increased apoptosis and changes in the tumor microenvironment (TME) that favored anti-tumor immunity .
Case Studies
- Case Study 1 : A study involving patient-derived organoids (PDOs) demonstrated that treatment with inhibitor 8 led to significant tumor size reduction and enhanced immune cell infiltration, particularly CD8+ T cells . This suggests that the inhibitor not only targets tumor cells directly but also modulates the TME to support immune responses.
- Case Study 2 : In another investigation, combining inhibitor 8 with immune checkpoint blockade (ICB) therapies showed synergistic effects, enhancing overall survival rates in preclinical models of PDAC . The combination therapy was particularly effective against tumors exhibiting resistance to single-agent treatments.
Data Table: Summary of Efficacy Findings
Challenges and Future Directions
Despite promising results, challenges remain in the clinical translation of KRAS G12D inhibitors. Resistance mechanisms, such as upregulation of alternative signaling pathways (e.g., NRAS and HRAS), have been observed following treatment with inhibitor 8 . Further research is needed to understand these mechanisms and develop combination therapies that can overcome resistance.
Properties
Molecular Formula |
C32H38BrFN6O2 |
---|---|
Molecular Weight |
637.6 g/mol |
IUPAC Name |
5-bromo-4-[4-(3,8-diazabicyclo[3.2.1]octan-3-yl)-2-[[1-[[(3R)-3-fluoropyrrolidin-1-yl]methyl]cyclopropyl]methoxy]-6,8-dihydro-5H-pyrido[3,4-d]pyrimidin-7-yl]naphthalen-2-ol |
InChI |
InChI=1S/C32H38BrFN6O2/c33-26-3-1-2-20-12-24(41)13-28(29(20)26)39-11-7-25-27(17-39)36-31(37-30(25)40-15-22-4-5-23(16-40)35-22)42-19-32(8-9-32)18-38-10-6-21(34)14-38/h1-3,12-13,21-23,35,41H,4-11,14-19H2/t21-,22?,23?/m1/s1 |
InChI Key |
WVLFHNZKPFLBQZ-DDRJZQQSSA-N |
Isomeric SMILES |
C1CN(C[C@@H]1F)CC2(CC2)COC3=NC4=C(CCN(C4)C5=C6C(=CC(=C5)O)C=CC=C6Br)C(=N3)N7CC8CCC(C7)N8 |
Canonical SMILES |
C1CC2CN(CC1N2)C3=NC(=NC4=C3CCN(C4)C5=C6C(=CC(=C5)O)C=CC=C6Br)OCC7(CC7)CN8CCC(C8)F |
Origin of Product |
United States |
Disclaimer and Information on In-Vitro Research Products
Please be aware that all articles and product information presented on BenchChem are intended solely for informational purposes. The products available for purchase on BenchChem are specifically designed for in-vitro studies, which are conducted outside of living organisms. In-vitro studies, derived from the Latin term "in glass," involve experiments performed in controlled laboratory settings using cells or tissues. It is important to note that these products are not categorized as medicines or drugs, and they have not received approval from the FDA for the prevention, treatment, or cure of any medical condition, ailment, or disease. We must emphasize that any form of bodily introduction of these products into humans or animals is strictly prohibited by law. It is essential to adhere to these guidelines to ensure compliance with legal and ethical standards in research and experimentation.