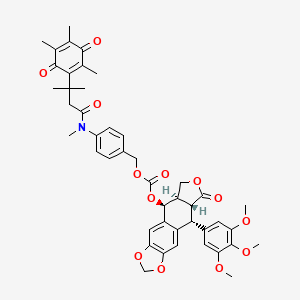
Antitumor agent-67
- Click on QUICK INQUIRY to receive a quote from our team of experts.
- With the quality product at a COMPETITIVE price, you can focus more on your research.
Overview
Description
Antitumor agent-67 is a potent antitumor compound known for its highly selective toxicity to cancer cells while causing minimal damage to normal cells . This compound has shown significant promise in preclinical studies, particularly in its ability to be activated by NAD(P)H:quinone oxidoreductase 1 (NQO1) and effectively liberate podophyllotoxin, a potent cytotoxic agent that kills tumor cells .
Preparation Methods
Synthetic Routes and Reaction Conditions: The synthesis of antitumor agent-67 involves multiple steps, starting from commercially available materials. The process typically includes the formation of key intermediates through a series of chemical reactions such as condensation, cyclization, and functional group modifications. Specific reaction conditions, including temperature, solvents, and catalysts, are optimized to achieve high yields and purity of the final product .
Industrial Production Methods: Industrial production of this compound may involve large-scale synthesis using batch or continuous flow reactors. The process is designed to ensure consistent quality and scalability. Techniques such as high-performance liquid chromatography (HPLC) and thin-layer chromatography (TLC) are employed for purification and quality control .
Chemical Reactions Analysis
Types of Reactions: Antitumor agent-67 undergoes various chemical reactions, including:
Oxidation: The compound can be oxidized to form reactive intermediates that contribute to its antitumor activity.
Reduction: Reduction reactions can activate the compound, enhancing its cytotoxic effects.
Substitution: Substitution reactions can modify the functional groups on the molecule, potentially altering its biological activity.
Common Reagents and Conditions: Common reagents used in these reactions include oxidizing agents like hydrogen peroxide, reducing agents such as sodium borohydride, and various catalysts to facilitate substitution reactions. Reaction conditions are carefully controlled to optimize the yield and selectivity of the desired products .
Major Products: The major products formed from these reactions include various derivatives of this compound, each with unique biological properties. These derivatives are often evaluated for their efficacy and safety in preclinical studies .
Scientific Research Applications
Antitumor agent-67 has a wide range of scientific research applications, including:
Chemistry: The compound is used as a model system to study the mechanisms of antitumor activity and to develop new synthetic methodologies.
Biology: Researchers use this compound to investigate cellular responses to cytotoxic agents and to identify potential biomarkers for cancer therapy.
Medicine: The compound is being explored as a potential therapeutic agent for various types of cancer, including liver, lung, and breast cancers.
Mechanism of Action
The mechanism of action of antitumor agent-67 involves its activation by NAD(P)H:quinone oxidoreductase 1 (NQO1), which leads to the release of podophyllotoxin. Podophyllotoxin exerts its cytotoxic effects by inhibiting topoisomerase II, an enzyme essential for DNA replication and cell division. This inhibition results in DNA damage, cell cycle arrest, and ultimately apoptosis of cancer cells . Additionally, this compound targets the mitochondrial pathways, leading to mitochondrial permeabilization and the release of pro-apoptotic factors .
Comparison with Similar Compounds
Podophyllotoxin: A natural product with potent antitumor activity, similar to the active form of antitumor agent-67.
Etoposide: A semisynthetic derivative of podophyllotoxin, used clinically for the treatment of various cancers.
Teniposide: Another podophyllotoxin derivative with similar mechanisms of action and clinical applications.
Uniqueness: this compound is unique in its selective activation by NAD(P)H:quinone oxidoreductase 1 (NQO1), which allows for targeted cytotoxicity towards cancer cells with high NQO1 expression. This selective activation reduces the potential for damage to normal cells, making it a promising candidate for cancer therapy .
Properties
Molecular Formula |
C45H47NO13 |
---|---|
Molecular Weight |
809.9 g/mol |
IUPAC Name |
[(5S,5aR,8aR,9R)-8-oxo-9-(3,4,5-trimethoxyphenyl)-5a,6,8a,9-tetrahydro-5H-[2]benzofuro[5,6-f][1,3]benzodioxol-5-yl] [4-[methyl-[3-methyl-3-(2,4,5-trimethyl-3,6-dioxocyclohexa-1,4-dien-1-yl)butanoyl]amino]phenyl]methyl carbonate |
InChI |
InChI=1S/C45H47NO13/c1-22-23(2)40(49)38(24(3)39(22)48)45(4,5)18-35(47)46(6)27-12-10-25(11-13-27)19-56-44(51)59-41-29-17-32-31(57-21-58-32)16-28(29)36(37-30(41)20-55-43(37)50)26-14-33(52-7)42(54-9)34(15-26)53-8/h10-17,30,36-37,41H,18-21H2,1-9H3/t30-,36+,37-,41+/m0/s1 |
InChI Key |
BWNLDDIKQLORJA-UDOLWIQMSA-N |
Isomeric SMILES |
CC1=C(C(=O)C(=C(C1=O)C)C(C)(C)CC(=O)N(C)C2=CC=C(C=C2)COC(=O)O[C@H]3[C@H]4COC(=O)[C@@H]4[C@@H](C5=CC6=C(C=C35)OCO6)C7=CC(=C(C(=C7)OC)OC)OC)C |
Canonical SMILES |
CC1=C(C(=O)C(=C(C1=O)C)C(C)(C)CC(=O)N(C)C2=CC=C(C=C2)COC(=O)OC3C4COC(=O)C4C(C5=CC6=C(C=C35)OCO6)C7=CC(=C(C(=C7)OC)OC)OC)C |
Origin of Product |
United States |
Disclaimer and Information on In-Vitro Research Products
Please be aware that all articles and product information presented on BenchChem are intended solely for informational purposes. The products available for purchase on BenchChem are specifically designed for in-vitro studies, which are conducted outside of living organisms. In-vitro studies, derived from the Latin term "in glass," involve experiments performed in controlled laboratory settings using cells or tissues. It is important to note that these products are not categorized as medicines or drugs, and they have not received approval from the FDA for the prevention, treatment, or cure of any medical condition, ailment, or disease. We must emphasize that any form of bodily introduction of these products into humans or animals is strictly prohibited by law. It is essential to adhere to these guidelines to ensure compliance with legal and ethical standards in research and experimentation.