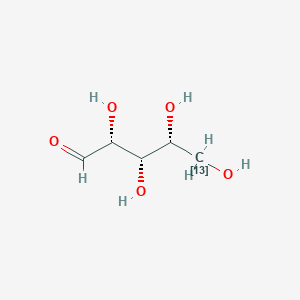
Xylose-5-13C
- Click on QUICK INQUIRY to receive a quote from our team of experts.
- With the quality product at a COMPETITIVE price, you can focus more on your research.
Overview
Description
Xylose-5-13C: is a stable isotope-labeled compound of xylose, a five-carbon sugar (aldopentose) commonly found in nature. The “13C” label indicates that the carbon at the fifth position of the xylose molecule is the carbon-13 isotope, which is a non-radioactive, stable isotope of carbon. This labeling is particularly useful in various scientific research applications, including metabolic studies and nuclear magnetic resonance (NMR) spectroscopy .
Preparation Methods
Synthetic Routes and Reaction Conditions: The preparation of Xylose-5-13C typically involves the incorporation of the carbon-13 isotope into the xylose molecule. This can be achieved through chemical synthesis or biosynthetic methods. One common approach is to start with a carbon-13 labeled precursor and carry out a series of chemical reactions to introduce the labeled carbon into the xylose structure. The specific reaction conditions may vary depending on the method used, but they generally involve controlled environments to ensure the precise incorporation of the isotope .
Industrial Production Methods: Industrial production of this compound often involves the use of advanced biotechnological processes. Microorganisms such as yeast or bacteria can be genetically engineered to incorporate carbon-13 into their metabolic pathways, resulting in the production of labeled xylose. These microorganisms are cultivated in bioreactors under specific conditions to optimize the yield of this compound .
Chemical Reactions Analysis
Types of Reactions: Xylose-5-13C undergoes various chemical reactions, including oxidation, reduction, and isomerization. These reactions are essential for studying the metabolic pathways and biochemical processes involving xylose.
Common Reagents and Conditions:
Oxidation: this compound can be oxidized to xylonic acid using oxidizing agents such as nitric acid or potassium permanganate.
Reduction: Reduction of this compound can be achieved using reducing agents like sodium borohydride, resulting in the formation of xylitol.
Isomerization: this compound can be isomerized to xylulose using xylose isomerase enzyme.
Major Products:
Xylonic Acid: Formed through oxidation.
Xylitol: Formed through reduction.
Xylulose: Formed through isomerization.
Scientific Research Applications
Chemistry: Xylose-5-13C is widely used in NMR spectroscopy to study the structure and dynamics of carbohydrates. The carbon-13 label provides a distinct signal in NMR spectra, allowing researchers to track the behavior of the labeled carbon atom in various chemical environments .
Biology: In biological research, this compound is used to investigate metabolic pathways involving xylose. It helps in understanding how organisms metabolize xylose and the role of specific enzymes in these processes .
Medicine: this compound is used in diagnostic tests to assess the absorption and metabolism of xylose in the human body. It is also employed in studies related to diabetes and other metabolic disorders .
Industry: In the industrial sector, this compound is used in the production of biofuels and biochemicals. It helps in optimizing fermentation processes and improving the efficiency of biofuel production from lignocellulosic biomass .
Mechanism of Action
Xylose-5-13C exerts its effects by participating in metabolic pathways involving xylose. The carbon-13 label allows researchers to trace the fate of the labeled carbon atom through various biochemical reactions. In the oxido-reductase pathway, xylose is first reduced to xylitol by xylose reductase, and then xylitol is oxidized to xylulose by xylitol dehydrogenase. Xylulose is subsequently phosphorylated to xylulose-5-phosphate, which enters the pentose phosphate pathway .
Comparison with Similar Compounds
Xylitol-5-13C: A labeled form of xylitol, used in similar metabolic studies.
Xylulose-5-13C: A labeled form of xylulose, used to study isomerization reactions.
Glucose-6-13C: A labeled form of glucose, used in metabolic studies involving glucose pathways.
Uniqueness: Xylose-5-13C is unique due to its specific labeling at the fifth carbon position, which provides detailed insights into the metabolic fate of xylose. This specificity makes it particularly valuable in studies focused on the pentose phosphate pathway and other xylose-related metabolic processes .
Properties
Molecular Formula |
C5H10O5 |
---|---|
Molecular Weight |
151.12 g/mol |
IUPAC Name |
(2R,3S,4R)-2,3,4,5-tetrahydroxy(513C)pentanal |
InChI |
InChI=1S/C5H10O5/c6-1-3(8)5(10)4(9)2-7/h1,3-5,7-10H,2H2/t3-,4+,5+/m0/s1/i2+1 |
InChI Key |
PYMYPHUHKUWMLA-WHXAWSBCSA-N |
Isomeric SMILES |
[13CH2]([C@H]([C@@H]([C@H](C=O)O)O)O)O |
Canonical SMILES |
C(C(C(C(C=O)O)O)O)O |
Origin of Product |
United States |
Disclaimer and Information on In-Vitro Research Products
Please be aware that all articles and product information presented on BenchChem are intended solely for informational purposes. The products available for purchase on BenchChem are specifically designed for in-vitro studies, which are conducted outside of living organisms. In-vitro studies, derived from the Latin term "in glass," involve experiments performed in controlled laboratory settings using cells or tissues. It is important to note that these products are not categorized as medicines or drugs, and they have not received approval from the FDA for the prevention, treatment, or cure of any medical condition, ailment, or disease. We must emphasize that any form of bodily introduction of these products into humans or animals is strictly prohibited by law. It is essential to adhere to these guidelines to ensure compliance with legal and ethical standards in research and experimentation.