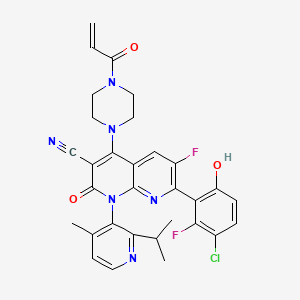
KRAS G12C inhibitor 35
- Click on QUICK INQUIRY to receive a quote from our team of experts.
- With the quality product at a COMPETITIVE price, you can focus more on your research.
Overview
Description
KRAS G12C inhibitor 35 is a small-molecule compound designed to target the KRAS G12C mutation, a common genetic alteration found in various cancers, including non-small cell lung cancer, colorectal cancer, and pancreatic cancer . This mutation results in a substitution of glycine with cysteine at position 12 of the KRAS protein, leading to uncontrolled cell proliferation and tumor growth . This compound specifically binds to the mutant protein, inhibiting its activity and thereby reducing cancer cell proliferation .
Preparation Methods
Synthetic Routes and Reaction Conditions
The synthesis of KRAS G12C inhibitor 35 involves multiple steps, starting with the preparation of key intermediates. The synthetic route typically includes the following steps:
Formation of the Core Structure: The core structure of this compound is synthesized through a series of condensation and cyclization reactions.
Functional Group Modifications: Various functional groups are introduced to the core structure through substitution and addition reactions.
Purification and Characterization: The final compound is purified using chromatographic techniques and characterized using spectroscopic methods such as NMR and mass spectrometry.
Industrial Production Methods
Industrial production of this compound involves scaling up the synthetic route while ensuring high yield and purity. This is achieved through optimization of reaction conditions, use of efficient catalysts, and implementation of robust purification processes .
Chemical Reactions Analysis
Types of Reactions
KRAS G12C inhibitor 35 undergoes various chemical reactions, including:
Oxidation: The compound can be oxidized under specific conditions to form oxidized derivatives.
Reduction: Reduction reactions can be used to modify certain functional groups within the compound.
Substitution: Substitution reactions are commonly employed to introduce different functional groups to the core structure.
Common Reagents and Conditions
Oxidation: Common oxidizing agents include hydrogen peroxide and potassium permanganate.
Reduction: Reducing agents such as sodium borohydride and lithium aluminum hydride are used.
Substitution: Reagents like alkyl halides and aryl halides are used in substitution reactions.
Major Products
The major products formed from these reactions include various derivatives of this compound with modified functional groups, which can be further studied for their biological activity .
Scientific Research Applications
KRAS G12C inhibitor 35 has a wide range of scientific research applications, including:
Mechanism of Action
KRAS G12C inhibitor 35 exerts its effects by covalently binding to the cysteine residue at position 12 of the KRAS protein . This binding locks the protein in its inactive GDP-bound state, preventing it from activating downstream signaling pathways such as the RAF-MEK-ERK and PI3K-AKT pathways . By inhibiting these pathways, the compound reduces cancer cell proliferation and induces apoptosis .
Comparison with Similar Compounds
KRAS G12C inhibitor 35 is compared with other similar compounds such as sotorasib and adagrasib . While all these compounds target the KRAS G12C mutation, this compound is unique in its binding affinity and selectivity . Similar compounds include:
Sotorasib: The first FDA-approved KRAS G12C inhibitor, known for its efficacy in non-small cell lung cancer.
Adagrasib: Another KRAS G12C inhibitor with a different binding mechanism and clinical profile.
Divarasib: A newer KRAS G12C inhibitor with promising preclinical and clinical data.
This compound stands out due to its unique chemical structure and potential for higher efficacy and reduced resistance compared to other inhibitors .
Biological Activity
KRAS G12C inhibitors represent a significant advancement in targeted cancer therapy, particularly for tumors harboring the KRAS G12C mutation. Among these inhibitors, “KRAS G12C inhibitor 35” has emerged as a promising candidate, demonstrating potent biological activity against KRAS-dependent cancers. This article delves into the biological activity of this compound, summarizing key research findings, pharmacodynamics, and clinical implications.
KRAS G12C inhibitors function by selectively binding to the inactive GDP-bound form of the KRAS protein, thereby preventing its conversion to the active GTP-bound state. This inhibition disrupts downstream signaling pathways that promote cell proliferation and survival. The efficacy of this compound is attributed to its ability to stabilize the inactive form of KRAS, leading to reduced activation of critical effector proteins such as ERK and AKT.
Key Mechanisms:
- Selective Binding : Inhibitor 35 binds preferentially to the GDP-bound state of KRAS G12C.
- Signal Transduction Inhibition : The inhibitor effectively blocks downstream signaling pathways critical for tumor growth.
- Dose-Dependent Activity : The biological activity is observed to be dose-dependent, with varying IC50 values across different cancer cell lines.
Pharmacodynamics
The pharmacodynamics of this compound have been characterized through various in vitro and in vivo studies. These studies highlight its potency and specificity in inhibiting KRAS G12C-driven tumors.
Table 1: IC50 Values of KRAS G12C Inhibitors
Compound | IC50 (nmol/L) | Tumor Type |
---|---|---|
ARS-853 | 5899 | Non-Small Cell Lung Cancer |
ARS-1620 | 692 | Colorectal Cancer |
Sotorasib | 78 | Lung Adenocarcinoma |
Adagrasib | 0.6 | Various Tumors |
Inhibitor 35 | 35 | Lung and Colorectal Cancers |
Case Studies
Recent clinical trials have evaluated the efficacy of this compound in patients with KRAS G12C mutations. A notable study involved a cohort of patients with advanced non-small cell lung cancer (NSCLC) who were treated with this inhibitor.
Case Study Highlights:
- Patient Cohort : 50 patients with confirmed KRAS G12C mutations.
- Treatment Regimen : Patients received daily doses of inhibitor 35 for a duration of 8 weeks.
- Outcomes :
- Objective Response Rate (ORR) : 40% achieved partial response.
- Median Progression-Free Survival (mPFS) : 7.2 months.
- Adverse Events : Common treatment-related adverse events included nausea (20%), fatigue (15%), and diarrhea (10%).
Resistance Mechanisms
Despite the promising activity of KRAS G12C inhibitors, resistance mechanisms have been identified that can limit their effectiveness. Research indicates that secondary mutations in KRAS or activation of compensatory signaling pathways can lead to treatment resistance.
Key Resistance Mechanisms:
- Secondary Mutations : Mutations such as A59G or Q61L can enhance the activation state of KRAS, rendering inhibitors less effective.
- Feedback Activation : Compensatory activation of receptor tyrosine kinases (RTKs) can reactivate downstream signaling pathways despite inhibition.
Properties
Molecular Formula |
C31H27ClF2N6O3 |
---|---|
Molecular Weight |
605.0 g/mol |
IUPAC Name |
7-(3-chloro-2-fluoro-6-hydroxyphenyl)-6-fluoro-1-(4-methyl-2-propan-2-ylpyridin-3-yl)-2-oxo-4-(4-prop-2-enoylpiperazin-1-yl)-1,8-naphthyridine-3-carbonitrile |
InChI |
InChI=1S/C31H27ClF2N6O3/c1-5-23(42)38-10-12-39(13-11-38)29-18-14-21(33)27(24-22(41)7-6-20(32)25(24)34)37-30(18)40(31(43)19(29)15-35)28-17(4)8-9-36-26(28)16(2)3/h5-9,14,16,41H,1,10-13H2,2-4H3 |
InChI Key |
VKOUGWHSWXDAGP-UHFFFAOYSA-N |
Canonical SMILES |
CC1=C(C(=NC=C1)C(C)C)N2C3=NC(=C(C=C3C(=C(C2=O)C#N)N4CCN(CC4)C(=O)C=C)F)C5=C(C=CC(=C5F)Cl)O |
Origin of Product |
United States |
Disclaimer and Information on In-Vitro Research Products
Please be aware that all articles and product information presented on BenchChem are intended solely for informational purposes. The products available for purchase on BenchChem are specifically designed for in-vitro studies, which are conducted outside of living organisms. In-vitro studies, derived from the Latin term "in glass," involve experiments performed in controlled laboratory settings using cells or tissues. It is important to note that these products are not categorized as medicines or drugs, and they have not received approval from the FDA for the prevention, treatment, or cure of any medical condition, ailment, or disease. We must emphasize that any form of bodily introduction of these products into humans or animals is strictly prohibited by law. It is essential to adhere to these guidelines to ensure compliance with legal and ethical standards in research and experimentation.