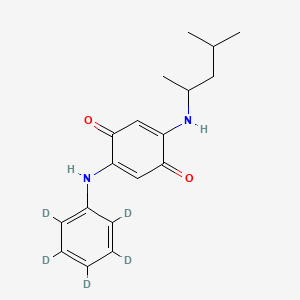
6PPD-quinone-d5
- Click on QUICK INQUIRY to receive a quote from our team of experts.
- With the quality product at a COMPETITIVE price, you can focus more on your research.
Overview
Description
6PPD-quinone-d5 (C₁₈H₁₇D₅N₂O₂; molecular weight 303.41 g/mol) is a deuterated isotopologue of the tire-derived transformation product 6PPD-quinone (C₁₈H₂₂N₂O₂; molecular weight 298.39 g/mol). It is synthesized by substituting five hydrogen atoms in the aniline ring of 6PPD-quinone with deuterium (²H) during synthesis, using benzene-d5 as the starting material . This compound serves as a critical internal standard in analytical chemistry, particularly for quantifying 6PPD-quinone in environmental and biological matrices via isotope dilution mass spectrometry . Its structural similarity to 6PPD-quinone ensures matched chromatographic behavior, while isotopic labeling enables unambiguous differentiation in mass spectrometry .
Preparation Methods
Synthetic Routes and Reaction Conditions
The synthesis of 6PPD-quinone-d5 typically involves the deuteration of 6PPD-quinone. The process begins with the oxidation of 6PPD to form 6PPD-quinone, which is then subjected to deuteration using deuterated reagents under controlled conditions . The reaction conditions often include the use of deuterated solvents and catalysts to ensure the incorporation of deuterium atoms into the quinone structure.
Industrial Production Methods
Industrial production of this compound follows similar synthetic routes but on a larger scale. The process involves the use of industrial-grade deuterated reagents and solvents, along with optimized reaction conditions to achieve high yields and purity . The production process is designed to be efficient and cost-effective, ensuring the availability of this compound for various research and industrial applications.
Chemical Reactions Analysis
Types of Reactions
6PPD-quinone-d5 undergoes several types of chemical reactions, including:
Oxidation: The compound can be further oxidized to form various oxidation products.
Reduction: Reduction reactions can convert this compound back to its precursor, 6PPD.
Substitution: The quinone structure allows for substitution reactions, where different functional groups can be introduced.
Common Reagents and Conditions
Common reagents used in these reactions include oxidizing agents like hydrogen peroxide and reducing agents such as sodium borohydride. The reactions are typically carried out under controlled temperature and pH conditions to ensure the desired products are formed .
Major Products Formed
The major products formed from these reactions include various oxidation and reduction products, as well as substituted quinones. These products are often analyzed using techniques like liquid chromatography-mass spectrometry (LC-MS) to determine their structure and properties .
Scientific Research Applications
Environmental Monitoring and Analysis
6PPD-quinone-d5 is primarily utilized as an internal standard in analytical chemistry for quantifying the presence of 6PPD-quinone in environmental samples. Its applications include:
- Water Quality Assessment : The compound is used in direct injection methods for analyzing water samples, achieving limits of quantitation in the mid-ng/L range. For instance, a study demonstrated that this method could detect 6PPD-quinone concentrations as low as 120 ng/L in real-world runoff samples .
- Method Development : The U.S. Environmental Protection Agency (EPA) has developed Method 1634 for determining 6PPD-quinone in aqueous matrices using liquid chromatography-tandem mass spectrometry (LC/MS/MS). This method has been validated for various water types, including stormwater and surface water .
Toxicological Studies
Research on the toxicity of this compound has been critical in understanding its ecological risks:
- Aquatic Toxicity : Studies have shown that exposure to 6PPD-quinone can inhibit growth and reproduction in freshwater organisms such as Daphnia pulex. Concentrations as low as 0.1 µg/L have been linked to adverse effects on growth . Additionally, juvenile coho salmon have demonstrated acute sensitivity, with median lethal concentrations (LC50) reported at around 0.04 µg/L .
- Chronic Effects : Long-term exposure studies indicate that even sublethal concentrations can lead to significant changes in behavior and reproductive capabilities in aquatic species, emphasizing the need for ongoing toxicological assessments .
Case Studies and Research Findings
Several case studies highlight the environmental impact and analytical methodologies involving this compound:
Mechanism of Action
The mechanism of action of 6PPD-quinone-d5 involves its interaction with molecular targets in aquatic organisms. The compound exerts its toxic effects through the formation of reactive oxygen species (ROS) and the disruption of cellular processes . The primary molecular targets include enzymes involved in oxidative stress response and cellular respiration . The pathways involved in its mechanism of action are related to oxidative stress and apoptosis, leading to cellular damage and death .
Comparison with Similar Compounds
Comparison with Structurally Similar Compounds
Structural Analogues
6PPD-Quinone
- Structure: Contains a dimethylbutyl group (N-(1,3-dimethylbutyl)) and a phenyl group (N′-phenyl) attached to a benzoquinone backbone.
- Properties : LogKow = 3.98, lipophilic, and persistent in environmental matrices such as water, air, and soil .
- Role : A toxic environmental contaminant linked to acute mortality in aquatic species (e.g., coho salmon) .
CPPD-Quinone
- Structure: Features a cyclohexyl group (N-cyclohexyl) instead of the dimethylbutyl group in 6PPD-quinone.
- Synthesis : Produced via similar oxidative pathways but with distinct starting materials (cyclohexylamine derivatives) .
- Toxicity: Limited data, but structural differences suggest altered bioactivity compared to 6PPD-quinone.
DTPD-Quinone and TPPD-Quinone
- DTPD-Quinone: Contains ditolyl (dimethylphenyl) substituents.
- TPPD-Quinone: Combines tolyl and phenyl groups.
- Environmental Presence: Detected in tire wear particles and urban runoff but at lower concentrations than 6PPD-quinone .
Isotopic Analogues
6PPD-Quinone-d5
- Key Features: Identical structure to 6PPD-quinone except for deuterium substitution in the aniline ring. Molecular weight difference (Δ = +5 Da) enables precise quantification via HRMS or LC-MS/MS .
- Analytical Advantages :
Other Isotopologues
- ¹³C-Labeled 6PPD-Quinone: Less common due to higher synthesis costs but offers similar utility in mass spectrometry.
Physicochemical and Analytical Comparisons
Table 1. Key Properties of this compound and Analogues
Chromatographic and Spectroscopic Behavior
- Retention Time: this compound co-elutes with 6PPD-quinone in reversed-phase LC (e.g., C18 columns) due to identical hydrophobicity .
- Mass Spectrometry: 6PPD-quinone: Base peak at m/z 299.2 ([M+H]⁺). this compound: Base peak at m/z 304.2 ([M+H]⁺), with fragmentation patterns mirroring the non-deuterated form .
Environmental and Toxicological Considerations
- Acute Toxicity: LC₅₀ values for fish (e.g., Oncorhynchus kisutch) range from 0.1–1.0 μg/L . Chirality: S-6PPD-quinone exhibits 10-fold greater toxicity than the R-form .
- This compound: No reported toxicity; used to correct for recovery losses during 6PPD-quinone quantification .
Biological Activity
6PPD-quinone-d5 is a derivative of 6PPD (N-(1,3-dimethylbutyl)-N'-phenyl-p-phenylenediamine), a widely used antioxidant in tire manufacturing. The transformation of 6PPD into 6PPD-quinone occurs through environmental oxidation processes, leading to significant ecological concerns due to its toxicological effects on aquatic organisms, particularly fish. This article explores the biological activity of this compound, focusing on its toxicity mechanisms, effects on fish species, and implications for environmental health.
Overview of this compound
This compound is a stable isotope-labeled version of 6PPD-quinone, which aids in tracing and quantifying the compound in biological and environmental studies. Understanding its biological activity is critical as it has been implicated in mass mortality events among salmonids in urban streams.
Mechanisms of Toxicity
Research indicates that exposure to 6PPD-quinone leads to significant dysregulation of genomic pathways associated with endothelial permeability and cell-cell adhesion, which may result in blood-brain barrier disruption. This disruption is particularly concerning for developing coho salmon embryos, where exposure has been linked to increased mortality and developmental abnormalities.
Key Findings:
- Acute Toxicity : Studies show that even low concentrations of 6PPD-quinone can induce acute mortality in various fish species, including coho salmon and brook trout. For instance, exposure to concentrations as low as 0.72 μg/L has demonstrated significant effects on survival rates and physiological responses such as increased blood glucose levels and hematocrit values .
- Developmental Impact : The compound affects critical developmental pathways, including those involved in muscle development and blood vessel formation. In laboratory settings, embryos exposed to environmentally relevant concentrations exhibited high rates of mortality and impaired growth .
Case Study 1: Coho Salmon
A study conducted on coho salmon embryos exposed to 6PPD-quinone revealed that significant mortality occurred at concentrations as low as 7.22 μg/L. The study utilized a pulse exposure method to simulate stormwater runoff conditions typical during spawning seasons. Results indicated that only 21% of embryos survived through hatching after multiple exposures .
Case Study 2: Brook Trout and Rainbow Trout
In another study assessing the effects on brook trout and rainbow trout, researchers found that exposure to varying concentrations of 6PPD-quinone resulted in behavioral changes indicative of respiratory distress, such as gasping and erratic swimming patterns. The median lethal concentrations (LC50) were determined for both species over different exposure durations, highlighting the compound's acute toxicity across different life stages .
Data Tables
The following table summarizes key findings from various studies regarding the toxicity levels of 6PPD-quinone across different fish species:
Environmental Implications
The presence of 6PPD-quinone in urban waterways poses significant risks not only to fish populations but also to broader aquatic ecosystems. Given its potent toxicity as demonstrated in various studies, regulatory assessments are necessary to mitigate its impact on biodiversity.
Q & A
Basic Research Questions
Q. What methodological steps are critical for quantifying 6PPD-quinone-d5 in aqueous matrices using isotope dilution?
- Use liquid chromatography-tandem mass spectrometry (LC-MS/MS) with deuterated this compound as an internal standard to correct for matrix effects and instrument variability. Calibrate the system with a 5–7 point curve spanning the expected concentration range. Validate the method by assessing precision (≤20% RSD), accuracy (80–120% recovery), and detection limits (e.g., ML of 0.1–1.0 µg/L). Follow EPA Method 1634 for sample preparation, including solid-phase extraction (SPE) and quality control (QC) protocols such as blanks and spiked duplicates .
Q. How should researchers design a literature review to assess 6PPD-quinone’s environmental toxicity?
- Conduct a systematic search across PubMed, ScienceDirect, and Google Scholar using keywords: 6PPD-Q, aquatic toxicity, bioaccumulation, and environmental transformation. Filter for peer-reviewed studies in English (post-2020). Extract data on LC50/EC50 values, test species (e.g., Oncorhynchus kisutch), and exposure durations. Cross-reference with regulatory reports (e.g., EPA Method 1634 validation studies) to identify data gaps, such as chronic toxicity in terrestrial ecosystems .
Q. What safety protocols are essential when handling this compound in laboratory settings?
- Use nitrile gloves, safety goggles, and lab coats to prevent dermal contact. Work in a fume hood to avoid inhalation. Store the compound at 4°C in airtight containers. In case of spills, decontaminate with absorbent materials (e.g., vermiculite) and dispose of waste as hazardous organic material. Regularly monitor air quality and maintain records of exposure incidents .
Advanced Research Questions
Q. How can researchers validate modifications to EPA Method 1634 for this compound analysis in complex matrices (e.g., soil or biota)?
- Perform a side-by-side comparison of the modified and original methods using spiked soil/biota samples. Assess labeled compound recovery (target: 70–130%), precision (≤15% RSD), and matrix effects. Document all changes (e.g., SPE sorbent type, gradient elution parameters) and submit data to EPA for equivalence approval. Include instrument raw data (e.g., chromatograms, peak areas) and QC metrics (calibration verification, blanks) in validation reports .
Q. What experimental designs address discrepancies in reported acute toxicity of 6PPD-quinone across studies?
- Replicate conflicting studies under controlled conditions, standardizing variables like water hardness (e.g., 50–100 mg/L CaCO3), pH (6.5–8.5), and dissolved oxygen. Use juvenile salmonids (e.g., O. mykiss) for comparability. Apply statistical tools (ANOVA, post-hoc tests) to identify confounding factors. Cross-validate findings with computational toxicology models (e.g., ECOSAR) to predict species sensitivity .
Q. How can isotopic tracing with this compound elucidate metabolic pathways in human hepatic models?
- Incubate this compound with human liver microsomes (HLMs) or HepG2 cells. Use high-resolution LC-HRMS to identify phase I/II metabolites (e.g., hydroxylated or glutathione conjugates). Compare retention times and fragmentation patterns with non-deuterated analogs to confirm structures. Quantify metabolite formation kinetics (Vmax, Km) and assess enzyme inhibition via CYP isoform-specific probes .
Q. What strategies improve detection limits for this compound in low-concentration environmental samples?
- Pre-concentrate samples using large-volume injection (e.g., 100 µL) or online SPE coupled with LC-MS/MS. Optimize ion source parameters (e.g., capillary voltage: 3.5 kV; desolvation temperature: 500°C) to enhance signal-to-noise ratios. Validate method sensitivity via repeated analysis of fortified samples at sub-ng/L levels, ensuring precision ≤25% and accuracy within 60–140% .
Q. Data Analysis and Interpretation
Q. How should researchers reconcile conflicting bioaccumulation data for 6PPD-quinone in terrestrial vs. aquatic species?
- Conduct species-specific bioconcentration factor (BCF) assays under standardized OECD 305 guidelines. Compare lipid-normalized concentrations in tissues (e.g., liver, gills) using isotope dilution. Apply probabilistic models to account for trophic transfer differences. Publish raw data (e.g., GC-MS/MS chromatograms, calibration curves) in open-access repositories for independent validation .
Q. What statistical approaches are optimal for analyzing dose-response relationships in 6PPD-quinone toxicity studies?
- Fit data to log-logistic or probit models using software like R (drc package) or GraphPad Prism. Report EC50 values with 95% confidence intervals. Assess goodness-of-fit via residual plots and AIC/BIC criteria. For non-monotonic responses, apply threshold models or Bayesian hierarchical frameworks .
Q. Tables
Key Analytical Parameters for this compound (EPA Method 1634) |
---|
Detection Limit (ML) |
Calibration Range |
Recovery (Spiked Samples) |
Precision (Intra-day RSD) |
Column Type |
Ionization Mode |
Reference |
Toxicity Data Variability in Aquatic Species |
---|
Species |
-------------------------- |
Oncorhynchus kisutch |
Daphnia magna |
Reference |
Properties
Molecular Formula |
C18H22N2O2 |
---|---|
Molecular Weight |
303.4 g/mol |
IUPAC Name |
2-(4-methylpentan-2-ylamino)-5-(2,3,4,5,6-pentadeuterioanilino)cyclohexa-2,5-diene-1,4-dione |
InChI |
InChI=1S/C18H22N2O2/c1-12(2)9-13(3)19-15-10-18(22)16(11-17(15)21)20-14-7-5-4-6-8-14/h4-8,10-13,19-20H,9H2,1-3H3/i4D,5D,6D,7D,8D |
InChI Key |
UBMGKRIXKUIXFQ-UPKDRLQUSA-N |
Isomeric SMILES |
[2H]C1=C(C(=C(C(=C1[2H])[2H])NC2=CC(=O)C(=CC2=O)NC(C)CC(C)C)[2H])[2H] |
Canonical SMILES |
CC(C)CC(C)NC1=CC(=O)C(=CC1=O)NC2=CC=CC=C2 |
Origin of Product |
United States |
Disclaimer and Information on In-Vitro Research Products
Please be aware that all articles and product information presented on BenchChem are intended solely for informational purposes. The products available for purchase on BenchChem are specifically designed for in-vitro studies, which are conducted outside of living organisms. In-vitro studies, derived from the Latin term "in glass," involve experiments performed in controlled laboratory settings using cells or tissues. It is important to note that these products are not categorized as medicines or drugs, and they have not received approval from the FDA for the prevention, treatment, or cure of any medical condition, ailment, or disease. We must emphasize that any form of bodily introduction of these products into humans or animals is strictly prohibited by law. It is essential to adhere to these guidelines to ensure compliance with legal and ethical standards in research and experimentation.