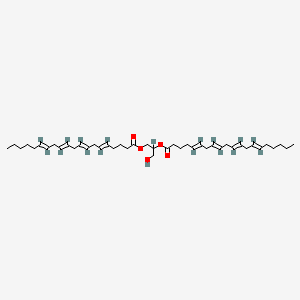
Diarachidonyl diglyceride
Overview
Description
. It is a significant lipid molecule involved in various biological processes, particularly in the signaling pathways related to inflammation and cellular responses.
Mechanism of Action
Target of Action
Diarachidonyl diglyceride, also known as Diarachidonin, primarily targets Protein Kinase C (PKC) and Protein Kinase D (PKD) isoforms . These kinases can sense diacylglycerol (DAG), a class of compounds to which this compound belongs . PKC and PKD play a crucial role in the regulation of metabolic homeostasis and might serve as targets for the treatment of metabolic disorders such as obesity and diabetes .
Mode of Action
This compound exerts its function through direct binding to its target proteins, characterized by the presence in their sequences of at least one conserved 1 (C1) domain . This interaction leads to the activation of PKC and PKD isoforms, which are involved in various physiological processes .
Biochemical Pathways
This compound is involved in various metabolic pathways in the cell. It is an important component of membranes that also acts as a secondary messenger . It is involved in multiple processes and pathways, such as protein transport, vesicle secretion, insulin signaling, cell growth, and proliferation .
Result of Action
The activation of PKC and PKD isoforms by this compound disrupts metabolic homeostasis, leading to the development of metabolic diseases such as diabetes and obesity . Aberrant activation of these kinases contributes to the development of these diseases .
Action Environment
The action of this compound can be influenced by various environmental factors. For example, the presence of nonsteroidal anti-inflammatory drugs stimulates the formation of this unique diglyceride . .
Biochemical Analysis
Biochemical Properties
Diarachidonin plays a crucial role in biochemical reactions, particularly in lipid signaling pathways. It interacts with several enzymes, proteins, and other biomolecules. One of the primary interactions is with phospholipase C, which hydrolyzes phosphatidylinositol 4,5-bisphosphate to produce diarachidonin and inositol trisphosphate. Diarachidonin is also a substrate for diacylglycerol lipase, which converts it into arachidonic acid, a precursor for eicosanoids such as prostaglandins and leukotrienes .
Cellular Effects
Diarachidonin influences various cellular processes, including cell signaling pathways, gene expression, and cellular metabolism. It acts as a secondary messenger in signal transduction pathways, particularly those involving protein kinase C. Diarachidonin can modulate the activity of protein kinase C, leading to changes in gene expression and cellular responses. Additionally, it has been shown to stimulate the production of prostaglandin E2 in rabbit kidney medulla slices, indicating its role in inflammatory responses .
Molecular Mechanism
At the molecular level, diarachidonin exerts its effects through binding interactions with specific biomolecules. It activates protein kinase C by increasing the concentration of diacylglycerol in the cell membrane. This activation leads to the phosphorylation of various target proteins, resulting in altered cellular functions. Diarachidonin also influences the activity of enzymes involved in the arachidonic acid pathway, such as cyclooxygenase and lipoxygenase, which are responsible for the production of eicosanoids .
Temporal Effects in Laboratory Settings
In laboratory settings, the effects of diarachidonin can change over time. The stability and degradation of diarachidonin are critical factors that influence its long-term effects on cellular function. Studies have shown that diarachidonin is relatively stable when stored at -20°C, with a shelf life of up to two years. Its effects on cellular function can vary depending on the duration of exposure and the specific experimental conditions .
Dosage Effects in Animal Models
The effects of diarachidonin vary with different dosages in animal models. At low doses, diarachidonin can stimulate cellular signaling pathways and promote cell proliferation. At high doses, it can have toxic effects, leading to cell death and tissue damage. Studies in animal models have shown that diarachidonin can induce inflammation and oxidative stress at high concentrations, highlighting the importance of dosage in its biological effects .
Metabolic Pathways
Diarachidonin is involved in several metabolic pathways, primarily those related to arachidonic acid metabolism. It is converted into arachidonic acid by diacylglycerol lipase, which is then further metabolized by cyclooxygenase and lipoxygenase to produce various eicosanoids. These eicosanoids play essential roles in inflammation, immune responses, and other physiological processes .
Transport and Distribution
Within cells and tissues, diarachidonin is transported and distributed through specific transporters and binding proteins. It is primarily localized in the cell membrane, where it interacts with membrane-bound enzymes and receptors. The distribution of diarachidonin can be influenced by factors such as lipid composition and membrane fluidity .
Subcellular Localization
Diarachidonin is predominantly localized in the cell membrane, where it exerts its effects on cellular signaling pathways. It can also be found in other subcellular compartments, such as the endoplasmic reticulum and mitochondria, where it may influence lipid metabolism and energy production. The subcellular localization of diarachidonin is regulated by specific targeting signals and post-translational modifications .
Preparation Methods
Synthetic Routes and Reaction Conditions: Diarachidonin can be synthesized from triarachidonin by treatment with lipase and purified by thin-layer chromatography . The solvent system used for purification is ligroin/ethyl ether/acetic acid (70:30:1), which allows the separation of 1,2- and 1,3-diacylglycerols from each other .
Industrial Production Methods: While specific industrial production methods for Diarachidonin are not extensively documented, the general approach involves enzymatic hydrolysis of triarachidonin followed by purification processes to achieve high purity levels .
Chemical Reactions Analysis
Types of Reactions: Diarachidonin undergoes various chemical reactions, including:
Oxidation: It can be oxidized to form hydroperoxides and other oxygenated derivatives.
Hydrolysis: Enzymatic hydrolysis can break down Diarachidonin into monoarachidonin and free arachidonic acid.
Common Reagents and Conditions:
Oxidation: Common oxidizing agents include molecular oxygen and peroxides.
Hydrolysis: Lipase enzymes are commonly used for hydrolysis reactions.
Major Products:
Oxidation: Hydroperoxides and other oxygenated derivatives.
Hydrolysis: Monoarachidonin and free arachidonic acid.
Scientific Research Applications
Diarachidonin has several applications in scientific research:
Chemistry: It is used as a model compound to study lipid oxidation and hydrolysis reactions.
Medicine: Research on Diarachidonin contributes to understanding inflammatory diseases and developing anti-inflammatory drugs.
Industry: It is used in the production of lipid-based products and as a standard in lipid analysis.
Comparison with Similar Compounds
Diolein: Contains oleic acid instead of arachidonic acid.
Dilinolein: Contains linoleic acid instead of arachidonic acid.
1-Stearoyl-2-oleoyl diglyceride: Contains stearic acid and oleic acid.
Uniqueness: Diarachidonin is unique due to its specific fatty acid composition, which includes arachidonic acid at both positions. This composition makes it particularly effective in activating protein kinase C and participating in inflammatory signaling pathways .
Properties
IUPAC Name |
[3-hydroxy-2-[(5E,8E,11E,14E)-icosa-5,8,11,14-tetraenoyl]oxypropyl] (5E,8E,11E,14E)-icosa-5,8,11,14-tetraenoate | |
---|---|---|
Source | PubChem | |
URL | https://pubchem.ncbi.nlm.nih.gov | |
Description | Data deposited in or computed by PubChem | |
InChI |
InChI=1S/C43H68O5/c1-3-5-7-9-11-13-15-17-19-21-23-25-27-29-31-33-35-37-42(45)47-40-41(39-44)48-43(46)38-36-34-32-30-28-26-24-22-20-18-16-14-12-10-8-6-4-2/h11-14,17-20,23-26,29-32,41,44H,3-10,15-16,21-22,27-28,33-40H2,1-2H3/b13-11+,14-12+,19-17+,20-18+,25-23+,26-24+,31-29+,32-30+ | |
Source | PubChem | |
URL | https://pubchem.ncbi.nlm.nih.gov | |
Description | Data deposited in or computed by PubChem | |
InChI Key |
FVXRWZPVZULNCQ-UXMFVEJOSA-N | |
Source | PubChem | |
URL | https://pubchem.ncbi.nlm.nih.gov | |
Description | Data deposited in or computed by PubChem | |
Canonical SMILES |
CCCCCC=CCC=CCC=CCC=CCCCC(=O)OCC(CO)OC(=O)CCCC=CCC=CCC=CCC=CCCCCC | |
Source | PubChem | |
URL | https://pubchem.ncbi.nlm.nih.gov | |
Description | Data deposited in or computed by PubChem | |
Isomeric SMILES |
CCCCC/C=C/C/C=C/C/C=C/C/C=C/CCCC(=O)OCC(CO)OC(=O)CCC/C=C/C/C=C/C/C=C/C/C=C/CCCCC | |
Source | PubChem | |
URL | https://pubchem.ncbi.nlm.nih.gov | |
Description | Data deposited in or computed by PubChem | |
Molecular Formula |
C43H68O5 | |
Source | PubChem | |
URL | https://pubchem.ncbi.nlm.nih.gov | |
Description | Data deposited in or computed by PubChem | |
Molecular Weight |
665.0 g/mol | |
Source | PubChem | |
URL | https://pubchem.ncbi.nlm.nih.gov | |
Description | Data deposited in or computed by PubChem | |
CAS No. |
82231-61-6 | |
Record name | Diarachidonyl diglyceride | |
Source | ChemIDplus | |
URL | https://pubchem.ncbi.nlm.nih.gov/substance/?source=chemidplus&sourceid=0082231616 | |
Description | ChemIDplus is a free, web search system that provides access to the structure and nomenclature authority files used for the identification of chemical substances cited in National Library of Medicine (NLM) databases, including the TOXNET system. | |
Retrosynthesis Analysis
AI-Powered Synthesis Planning: Our tool employs the Template_relevance Pistachio, Template_relevance Bkms_metabolic, Template_relevance Pistachio_ringbreaker, Template_relevance Reaxys, Template_relevance Reaxys_biocatalysis model, leveraging a vast database of chemical reactions to predict feasible synthetic routes.
One-Step Synthesis Focus: Specifically designed for one-step synthesis, it provides concise and direct routes for your target compounds, streamlining the synthesis process.
Accurate Predictions: Utilizing the extensive PISTACHIO, BKMS_METABOLIC, PISTACHIO_RINGBREAKER, REAXYS, REAXYS_BIOCATALYSIS database, our tool offers high-accuracy predictions, reflecting the latest in chemical research and data.
Strategy Settings
Precursor scoring | Relevance Heuristic |
---|---|
Min. plausibility | 0.01 |
Model | Template_relevance |
Template Set | Pistachio/Bkms_metabolic/Pistachio_ringbreaker/Reaxys/Reaxys_biocatalysis |
Top-N result to add to graph | 6 |
Feasible Synthetic Routes
Q1: How does diarachidonin influence prostaglandin E2 production in the kidney?
A: Research indicates that diarachidonin effectively stimulates the production of prostaglandin E2 (PGE2) in rabbit kidney medulla slices []. This effect was observed to be dose-dependent, with higher concentrations of diarachidonin leading to a greater increase in PGE2 synthesis. Notably, diarachidonin's impact on PGE2 production was found to be significantly stronger than that of arachidonic acid, exceeding it by twofold at comparable concentrations [].
Q2: What is the proposed mechanism behind diarachidonin's effect on PGE2 synthesis?
A: The study suggests that diarachidonin's ability to stimulate PGE2 production is not reliant on the activation of phospholipase A2, including the phosphatidic acid-specific isoform []. This conclusion is drawn from the observation that EGTA, a calcium chelator known to inhibit phospholipase A2, did not hinder diarachidonin-induced PGE2 formation. Instead, the researchers propose an alternative mechanism: diarachidonin might be metabolized by diacylglycerol and monoacylglycerol lipases to release arachidonic acid, which then acts as a substrate for PGE2 synthesis []. This proposed pathway suggests a sequential action of lipases in mediating diarachidonin's effect on PGE2 production.
Q3: Does diarachidonin interact with calcium and phospholipid-dependent protein kinase?
A: Yes, diarachidonin demonstrates a significant ability to activate calcium and phospholipid-dependent protein kinase (protein kinase C) []. This activation is achieved through a unique mechanism: diarachidonin increases the enzyme's affinity for phospholipids and simultaneously decreases the required concentration of calcium ions (Ca2+) for activation, bringing it down to the micromolar range []. Interestingly, diarachidonin's efficacy in this activation is directly related to the presence of unsaturated fatty acids, particularly at the second position of its glycerol backbone. Diacylglycerols with unsaturated fatty acids like diolein, dilinolein, and diarachidonin itself showed the most potent activation []. This finding suggests a potential link between diarachidonin, protein kinase C activation, and the broader cellular process of phosphatidylinositol turnover.
Disclaimer and Information on In-Vitro Research Products
Please be aware that all articles and product information presented on BenchChem are intended solely for informational purposes. The products available for purchase on BenchChem are specifically designed for in-vitro studies, which are conducted outside of living organisms. In-vitro studies, derived from the Latin term "in glass," involve experiments performed in controlled laboratory settings using cells or tissues. It is important to note that these products are not categorized as medicines or drugs, and they have not received approval from the FDA for the prevention, treatment, or cure of any medical condition, ailment, or disease. We must emphasize that any form of bodily introduction of these products into humans or animals is strictly prohibited by law. It is essential to adhere to these guidelines to ensure compliance with legal and ethical standards in research and experimentation.