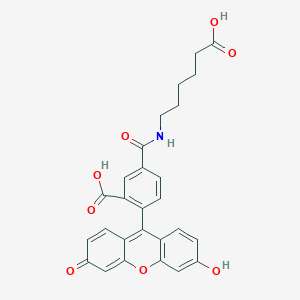
FluorX 5-isomer
Overview
Description
FluorX 5-isomer is a fluorescent dye derived from fluorescin. It is a xanthene dye with an absorption wavelength of 494 nm and an emission wavelength of 520 nm . This compound is used extensively in various scientific fields due to its fluorescent properties.
Mechanism of Action
Target of Action
It is commonly used as a fluorescein-based fluorescent label for proteins . This suggests that its primary targets could be proteins that can be analyzed in applications such as quenching experiments .
Mode of Action
The FluorX 5-isomer interacts with its targets (proteins) by covalently binding to them, thereby enabling their detection through fluorescence . The compound contains a fluorescein moiety, which is responsible for its fluorescent properties . When excited by light at a specific wavelength, the fluorescein moiety emits light at a different wavelength, which can be detected and measured .
Result of Action
The primary result of this compound’s action is the labeling of proteins, which allows for their detection and analysis via fluorescence . This can provide valuable information about the proteins’ structure, function, and interactions, contributing to our understanding of various biological processes and disease states.
Action Environment
The action, efficacy, and stability of this compound can be influenced by various environmental factors, including the pH and temperature of the solution, the presence of other molecules, and the specific properties of the target proteins . For instance, the fluorescence of the compound is typically measured in a solution with a specific pH .
Biochemical Analysis
Biochemical Properties
FluorX 5-isomer plays a crucial role in various biochemical reactions. It interacts with several enzymes, proteins, and other biomolecules, influencing their activity and function. One of the primary interactions of this compound is with the enzyme thymidylate synthase, which is involved in DNA synthesis. This compound inhibits the activity of thymidylate synthase by forming a stable complex with the enzyme, thereby preventing the synthesis of thymidine monophosphate, a nucleotide essential for DNA replication . Additionally, this compound interacts with other proteins involved in nucleotide metabolism, such as orotate phosphoribosyltransferase, which converts this compound into its active form .
Cellular Effects
This compound has profound effects on various types of cells and cellular processes. It influences cell function by affecting cell signaling pathways, gene expression, and cellular metabolism. In cancer cells, this compound induces DNA damage, leading to cell cycle arrest and apoptosis . It also modulates the expression of genes involved in DNA repair, cell cycle regulation, and apoptosis, such as p53, CDKN1A, and FAS . Furthermore, this compound affects cellular metabolism by inhibiting enzymes involved in nucleotide synthesis, leading to an imbalance in nucleotide pools and disruption of DNA and RNA synthesis .
Molecular Mechanism
The molecular mechanism of action of this compound involves several key steps. Upon entering the cell, this compound is converted into its active metabolites, such as fluorodeoxyuridine monophosphate and fluorouridine triphosphate . These metabolites inhibit the activity of thymidylate synthase and other enzymes involved in nucleotide synthesis, leading to the disruption of DNA and RNA synthesis . This compound also incorporates into DNA and RNA, causing DNA strand breaks and RNA dysfunction . Additionally, this compound modulates gene expression by affecting transcription factors and signaling pathways involved in cell cycle regulation and apoptosis .
Temporal Effects in Laboratory Settings
The effects of this compound change over time in laboratory settings. The stability and degradation of this compound are critical factors that influence its long-term effects on cellular function. In in vitro studies, this compound has been shown to degrade over time, leading to a decrease in its efficacy . In in vivo studies, this compound exhibits prolonged effects due to its slow degradation and sustained release from tissues . Long-term exposure to this compound can result in persistent DNA damage, cell cycle arrest, and apoptosis .
Dosage Effects in Animal Models
The effects of this compound vary with different dosages in animal models. At low doses, this compound exhibits minimal toxicity and effectively inhibits tumor growth by inducing DNA damage and apoptosis . At high doses, this compound can cause severe toxic effects, including bone marrow suppression, gastrointestinal toxicity, and hepatotoxicity . Threshold effects have been observed, where a certain dosage is required to achieve a therapeutic effect, and exceeding this dosage leads to adverse effects .
Metabolic Pathways
This compound is involved in several metabolic pathways, primarily related to nucleotide metabolism. It is converted into its active metabolites by the enzyme orotate phosphoribosyltransferase . These metabolites inhibit the activity of thymidylate synthase and other enzymes involved in nucleotide synthesis, leading to the disruption of DNA and RNA synthesis . This compound also affects metabolic flux by altering the levels of nucleotides and other metabolites involved in DNA and RNA synthesis .
Transport and Distribution
This compound is transported and distributed within cells and tissues through various mechanisms. It is taken up by cells via nucleoside transporters and distributed to different cellular compartments . This compound interacts with binding proteins and transporters that facilitate its localization and accumulation in specific tissues . The distribution of this compound within tissues is influenced by factors such as tissue perfusion, cellular uptake, and binding affinity to cellular components .
Subcellular Localization
The subcellular localization of this compound is critical for its activity and function. This compound is primarily localized in the nucleus, where it exerts its effects on DNA synthesis and gene expression . It is also found in the cytoplasm, where it interacts with RNA and other cytoplasmic components . The localization of this compound is influenced by targeting signals and post-translational modifications that direct it to specific compartments or organelles .
Preparation Methods
The synthesis of FluorX 5-isomer involves several steps. One common method includes the preparation of 5-aminofluorescein, which serves as a precursor. The synthesis of 5-aminofluorescein can be achieved through a series of reactions involving the nitration of fluorescein followed by reduction . Industrial production methods often involve the use of high-purity reagents and controlled reaction conditions to ensure the consistency and quality of the final product.
Chemical Reactions Analysis
FluorX 5-isomer undergoes various chemical reactions, including oxidation, reduction, and substitution. Common reagents used in these reactions include oxidizing agents like potassium permanganate and reducing agents such as sodium borohydride. The major products formed from these reactions depend on the specific conditions and reagents used. For example, oxidation of this compound can lead to the formation of quinone derivatives .
Scientific Research Applications
FluorX 5-isomer is widely used in scientific research due to its fluorescent properties. It is commonly used as a fluorescent probe in biological studies to label and visualize biomolecules such as proteins and nucleic acids . In chemistry, it is used in various analytical techniques, including fluorescence microscopy and flow cytometry. In medicine, this compound is used in diagnostic assays to detect specific biomarkers. Additionally, it has applications in environmental analysis to monitor pollutants and other chemical substances .
Comparison with Similar Compounds
FluorX 5-isomer is unique compared to other similar compounds due to its specific absorption and emission wavelengths. Similar compounds include other xanthene dyes such as rhodamine and fluorescein derivatives. These compounds also exhibit fluorescent properties but differ in their spectral characteristics and applications. For example, rhodamine dyes have different absorption and emission wavelengths, making them suitable for different types of fluorescence-based assays .
Similar Compounds
- Rhodamine
- Fluorescein derivatives
- Alexa Fluor dyes
- BODIPY dyes
Properties
CAS No. |
265981-56-4 |
---|---|
Molecular Formula |
C54H46N2O16 |
Molecular Weight |
978.9 g/mol |
IUPAC Name |
6-[(3',6'-dihydroxy-1-oxospiro[2-benzofuran-3,9'-xanthene]-5-carbonyl)amino]hexanoic acid;6-[(3',6'-dihydroxy-3-oxospiro[2-benzofuran-1,9'-xanthene]-5-carbonyl)amino]hexanoic acid |
InChI |
InChI=1S/2C27H23NO8/c29-16-6-9-20-22(13-16)35-23-14-17(30)7-10-21(23)27(20)19-8-5-15(12-18(19)26(34)36-27)25(33)28-11-3-1-2-4-24(31)32;29-16-6-9-19-22(13-16)35-23-14-17(30)7-10-20(23)27(19)21-12-15(5-8-18(21)26(34)36-27)25(33)28-11-3-1-2-4-24(31)32/h2*5-10,12-14,29-30H,1-4,11H2,(H,28,33)(H,31,32) |
InChI Key |
WEJBWDKWIFHWDE-UHFFFAOYSA-N |
SMILES |
C1=CC(=C(C=C1C(=O)NCCCCCC(=O)O)C(=O)O)C2=C3C=CC(=O)C=C3OC4=C2C=CC(=C4)O |
Canonical SMILES |
C1=CC2=C(C=C1C(=O)NCCCCCC(=O)O)C3(C4=C(C=C(C=C4)O)OC5=C3C=CC(=C5)O)OC2=O.C1=CC2=C(C=C1C(=O)NCCCCCC(=O)O)C(=O)OC23C4=C(C=C(C=C4)O)OC5=C3C=CC(=C5)O |
Origin of Product |
United States |
Retrosynthesis Analysis
AI-Powered Synthesis Planning: Our tool employs the Template_relevance Pistachio, Template_relevance Bkms_metabolic, Template_relevance Pistachio_ringbreaker, Template_relevance Reaxys, Template_relevance Reaxys_biocatalysis model, leveraging a vast database of chemical reactions to predict feasible synthetic routes.
One-Step Synthesis Focus: Specifically designed for one-step synthesis, it provides concise and direct routes for your target compounds, streamlining the synthesis process.
Accurate Predictions: Utilizing the extensive PISTACHIO, BKMS_METABOLIC, PISTACHIO_RINGBREAKER, REAXYS, REAXYS_BIOCATALYSIS database, our tool offers high-accuracy predictions, reflecting the latest in chemical research and data.
Strategy Settings
Precursor scoring | Relevance Heuristic |
---|---|
Min. plausibility | 0.01 |
Model | Template_relevance |
Template Set | Pistachio/Bkms_metabolic/Pistachio_ringbreaker/Reaxys/Reaxys_biocatalysis |
Top-N result to add to graph | 6 |
Feasible Synthetic Routes
Q1: Why is 6-[Fluorescein-5(6)-carboxamido]hexanoic acid incorporated into the silicone nanocomposites for LED encapsulation?
A1: FCHA is introduced into the silicone nanocomposites to enable color conversion. When excited by a blue LED at a wavelength around 455 nm, the FCHA undergoes secondary emission, producing yellow light at around 571 nm []. This allows for the creation of white light LEDs, which are in high demand for various lighting applications.
Q2: How is 6-[Fluorescein-5(6)-carboxamido]hexanoic acid integrated into the silicone matrix containing Zirconium dioxide (ZrO2) nanoparticles?
A2: FCHA is not directly incorporated into the silicone matrix. Instead, it is attached to ZrO2 nanoparticles that have been modified with polydimethylsiloxane (PDMS) brushes. This "grafting-to" approach, using a ligand exchange process, ensures homogenous dispersion of the FCHA within the silicone matrix, contributing to the nanocomposite's desirable optical properties [].
Disclaimer and Information on In-Vitro Research Products
Please be aware that all articles and product information presented on BenchChem are intended solely for informational purposes. The products available for purchase on BenchChem are specifically designed for in-vitro studies, which are conducted outside of living organisms. In-vitro studies, derived from the Latin term "in glass," involve experiments performed in controlled laboratory settings using cells or tissues. It is important to note that these products are not categorized as medicines or drugs, and they have not received approval from the FDA for the prevention, treatment, or cure of any medical condition, ailment, or disease. We must emphasize that any form of bodily introduction of these products into humans or animals is strictly prohibited by law. It is essential to adhere to these guidelines to ensure compliance with legal and ethical standards in research and experimentation.