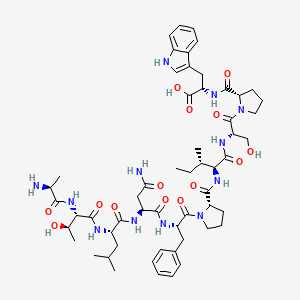
HIV-1, HIV-2 Protease Substrate
- Click on QUICK INQUIRY to receive a quote from our team of experts.
- With the quality product at a COMPETITIVE price, you can focus more on your research.
Overview
Description
HIV-1 and HIV-2 proteases are aspartyl proteases critical for viral maturation. Both enzymes are homodimers composed of two identical 99-amino acid monomers, facilitating cleavage of Gag and Gag-Pol polyproteins to produce functional viral proteins . Despite their structural and functional similarities, HIV-2 is less pathogenic and transmissible than HIV-1, with lower viral loads and slower disease progression . Sequence identity between the two proteases ranges from 39% to 48%, with key polymorphisms in substrate-binding regions influencing inhibitor efficacy and drug resistance . The substrate-binding sites differ by only four conservative substitutions: Val32Ile, Ile47Val, Leu76Met, and Val82Ile, which alter methyl group interactions but preserve overall substrate recognition .
Preparation Methods
Synthetic Routes and Reaction Conditions: The synthesis of HIV-1, HIV-2 protease substrates typically involves solid-phase peptide synthesis (SPPS), a method that allows for the sequential addition of amino acids to a growing peptide chain anchored to a solid resin . The process includes:
Deprotection: Removal of the protecting group from the amino terminus of the resin-bound amino acid.
Coupling: Addition of the next amino acid in the sequence, activated by coupling reagents such as N,N’-diisopropylcarbodiimide (DIC) and hydroxybenzotriazole (HOBt).
Cleavage: The final peptide is cleaved from the resin using a cleavage cocktail, typically containing trifluoroacetic acid (TFA).
Industrial Production Methods: Industrial production of HIV-1, HIV-2 protease substrates follows similar principles but on a larger scale, often utilizing automated peptide synthesizers to increase efficiency and yield .
Chemical Reactions Analysis
Types of Reactions: The HIV-1, HIV-2 protease substrate undergoes hydrolysis, a reaction where the peptide bond is cleaved by the HIV protease enzyme . This reaction is crucial for the maturation of the virus.
Common Reagents and Conditions:
Reagents: Water molecules and catalytic aspartate residues in the active site of the protease.
Conditions: Physiological conditions, typically at a pH of around 7.4 and body temperature (37°C).
Major Products: The hydrolysis of the this compound results in smaller peptide fragments, which are essential for the formation of mature viral proteins .
Scientific Research Applications
Drug Design and Inhibitor Development
Substrate Envelope Model
The substrate envelope model is a pivotal concept in designing potent inhibitors for HIV-1 protease. By confining inhibitors within the consensus volume of natural substrates, researchers have developed inhibitors that are less susceptible to resistance mutations. A study reported the design of inhibitors that effectively bind to multi-drug resistant variants of HIV-1 protease, demonstrating robust antiviral activity across different viral clades and drug-resistant strains . This model allows for the optimization of inhibitors by ensuring they fit well within the substrate envelope, minimizing non-substrate-like interactions that can lead to resistance .
Case Study: Darunavir Analogues
Research has shown that modifications to darunavir, a potent HIV-1 protease inhibitor, can enhance its binding affinity and efficacy against resistant strains. A panel of ten darunavir analogues was synthesized, exhibiting low enzymatic inhibition constants and high potency in cell culture assays. These modifications included changes at the P1' position of the inhibitor, which significantly improved interactions with the protease . The findings highlight the potential for creating inhibitors with a high genetic barrier to resistance.
Understanding Resistance Mechanisms
Structural Insights into Resistance
The intrinsic resistance of HIV-2 to many FDA-approved HIV-1 protease inhibitors has been attributed to specific amino acid variations in the protease structure. Studies have identified key residues in the ligand-binding pocket that confer this resistance. For instance, single amino acid changes in positions 32, 47, and 76 of HIV-2 protease can increase susceptibility to multiple inhibitors, demonstrating how structural variations influence drug efficacy .
Comparative Studies
Comparative structural studies between HIV-1 and HIV-2 proteases have revealed critical differences in substrate specificity and inhibitor binding. For example, while both proteases share significant sequence identity, their responses to inhibitors like amprenavir differ markedly due to variations in their active sites . These insights are crucial for developing effective treatments for both types of HIV.
Kinetic and Thermodynamic Studies
Specificity Engineering
Recent advancements in computational design have allowed for the engineering of HIV proteases with altered substrate specificity. A variant designed to preferentially bind a specific substrate exhibited a threefold increase in binding affinity compared to wild-type protease. This approach not only aids in understanding substrate interactions but also facilitates the development of targeted therapies .
Thermodynamic Analysis
Thermodynamic studies have provided valuable insights into the binding dynamics between proteases and their substrates or inhibitors. These studies reveal how conformational changes upon binding can affect enzyme activity and substrate processing, which is critical for understanding how inhibitors can be designed to effectively block protease function .
Structural Studies and Insights
Crystallography and Substrate Binding
Crystallographic studies have elucidated how various substrates interact with HIV-1 protease. By analyzing crystal structures of enzyme-substrate complexes, researchers have identified key interactions that stabilize binding and inform inhibitor design. The identification of conserved hydrogen bonding patterns between substrate analogs and protease residues is particularly important for developing new therapeutic agents .
Table: Key Findings from Structural Studies
Mechanism of Action
The HIV-1, HIV-2 protease substrate is cleaved by the HIV protease enzyme through a general acid-base mechanism involving two catalytic aspartate residues . The process includes:
Binding: The substrate binds to the active site of the protease, forming hydrogen bonds with the catalytic aspartates.
Catalysis: A water molecule, activated by the aspartates, attacks the carbonyl carbon of the peptide bond, leading to its cleavage.
Release: The resulting peptide fragments are released, completing the reaction.
Comparison with Similar Compounds
Comparison with Similar Compounds
Substrate Specificity
Both proteases cleave oligopeptide substrates representing Gag and Gag-Pol cleavage sites, but subtle differences exist (Table 1). HIV-1 prefers cleavage sites with hydrophobic or aromatic residues (e.g., -PhePro- or -TyrPro-) , while HIV-2 exhibits broader specificity. Early studies suggested significant divergence in substrate preferences , but later work revealed overlapping specificities due to conserved active-site architecture . For example:
- HIV-1 MA/CA cleavage site : HIV-1 protease cleaves Ser-Gln-Asn-Tyr*Pro-Ile-Val more efficiently than HIV-2 protease .
- HIV-2 CA/p2 cleavage site : HIV-2 protease processes Val-Leu-Ala-Met*Ser-Pro-Val at rates comparable to HIV-1 .
Structural analyses confirm that substrate shape, particularly the complementary fit to the protease’s S2/S2′ subsites, determines specificity .
Inhibitor Binding and Efficacy
Clinical inhibitors of HIV-1 protease (e.g., darunavir, lopinavir) show reduced potency against HIV-2 protease (Table 2). For instance:
- Darunavir : Inhibits HIV-1 protease with IC₅₀ ≈ 1 nM but requires 10–100× higher concentrations for HIV-2 .
- GRL-06579A: A darunavir analog, maintains sub-nanomolar inhibition for both proteases due to conserved hydrogen bonding .
Crystal structures reveal near-identical binding modes (RMSD = 1.1 Å for main-chain atoms), with conserved hydrogen bonds and water-mediated interactions . However, hydrophobic contacts (e.g., Val82 in HIV-1 vs. Ile82 in HIV-2) reduce inhibitor affinity in HIV-2 .
Structural and Mechanistic Differences
Key structural distinctions include:
- Flap Dynamics : HIV-1’s β-hairpin flaps (residues 45–55) exhibit greater flexibility, influencing substrate entry and drug resistance .
- Active-Site Cavity : HIV-2’s active site is marginally larger due to Ile47 replacing Val47, accommodating bulkier substrates .
- Dimer Stability : HIV-2 protease dimers dissociate more readily under physiological conditions, affecting inhibitor binding kinetics .
Drug Resistance Mechanisms
HIV-2 exhibits intrinsic resistance to non-nucleoside reverse transcriptase inhibitors (NNRTIs) and reduced susceptibility to protease inhibitors (PIs) due to natural polymorphisms . For example:
- V32I Mutation : Reduces darunavir binding in HIV-1 but has minimal impact in HIV-2 due to pre-existing isoleucine at position 32 .
HIV-2’s integrase also differs at 33% of residues in the catalytic domain, leading to unique resistance pathways against INSTIs (e.g., raltegravir) .
Data Tables
Table 1: Substrate Specificity Comparison
Table 2: Inhibitor Efficacy
Inhibitor | HIV-1 IC₅₀ (nM) | HIV-2 IC₅₀ (nM) | Reference |
---|---|---|---|
Darunavir | 1 | 50–100 | |
Saquinavir | 10 | 500 | |
GRL-98065 | 0.8 | 2.5 |
Biological Activity
HIV-1 and HIV-2 proteases are essential for the maturation of the viral particles, as they cleave the Gag and Gag-Pol polyproteins into functional proteins. Understanding the biological activity of their substrates is crucial for developing effective antiretroviral therapies. This article discusses the structural and kinetic characteristics of HIV-1 and HIV-2 protease substrates, their specificity, and the implications for drug resistance.
Structural Characteristics
HIV proteases are symmetrical homodimers composed of two identical 99-amino-acid subunits. They utilize an aspartic acid catalytic mechanism to cleave peptide bonds in their substrates. The substrate binding involves several key residues within the active site, which are critical for substrate specificity.
Substrate Binding Sites
The binding sites of HIV proteases include:
- P1 to P4 Positions : These positions correspond to specific amino acids in the substrate that interact with the protease.
- Hydrophobic Core : The core is formed by beta sheets, providing a stable environment for substrate binding.
The substrate envelope hypothesis suggests that effective inhibitors must fit within a specific volume defined by the native substrates, minimizing non-substrate-like interactions that could lead to drug resistance .
Kinetic Properties
Kinetic studies have shown that both HIV-1 and HIV-2 proteases exhibit distinct preferences for different substrate sequences.
Key Kinetic Parameters
- Km Values : Reflect the affinity of the enzyme for its substrate. Studies indicate that Km values for various peptides range from 0.07 to 0.57 mM across different protease variants .
- Catalytic Efficiency (kcat) : Represents the turnover number of the enzyme. For example, a fluorogenic substrate designed for HIV-1 protease demonstrated a kcat of 7.4 s−1 with a Km of 15 μM, highlighting its efficiency in cleavage assays .
Substrate | Protease Type | Km (mM) | kcat (s−1) |
---|---|---|---|
p2/NC | HIV-1 | 0.15 | 5.2 |
CA/p2 | HIV-2 | 0.12 | 4.8 |
RT–RH | Engineered Variant | 0.05 | 6.5 |
Substrate Specificity
The specificity of HIV proteases is influenced by several factors, including amino acid composition at key positions (P1, P2, etc.) and structural flexibility.
Differences Between HIV-1 and HIV-2
Research indicates that while both proteases share significant structural similarities, they differ in their cleavage site sequences and substrate preferences:
- HIV-1 Protease : More efficient with certain peptide substrates compared to HIV-2.
- HIV-2 Protease : Exhibits intrinsic resistance to many FDA-approved inhibitors due to differences in binding pocket residues .
Case Studies
One study engineered a variant of HIV protease (Pr3) with altered specificity that preferentially binds to one out of three natural substrates, demonstrating an increase in binding affinity by threefold compared to wild-type protease . This highlights the potential for designing targeted inhibitors based on substrate specificity.
Implications for Drug Resistance
Drug resistance mutations (DRMs) significantly affect the efficacy of protease inhibitors (PIs). Mutations at key residues can alter inhibitor binding without impacting substrate recognition:
- Common DRMs include substitutions at positions 32, 47, and 82 in HIV-1, which correlate with reduced susceptibility to various PIs .
- Structural analyses have shown that these mutations often occur at residues critical for maintaining substrate specificity, suggesting a complex relationship between drug resistance and natural substrate interactions .
Q & A
Basic Research Questions
Q. What experimental strategies are recommended for designing HIV protease substrates to study enzyme kinetics?
To design substrates for kinetic assays, researchers should:
- Analyze conserved binding residues : Both HIV-1 and HIV-2 proteases share four conserved substrate-binding residues, critical for maintaining substrate recognition .
- Leverage structural databases : Use the HIV Protease Database ( ) to compare protease-substrate complexes across strains, focusing on conserved hydrogen bonding patterns and hydrophobic interactions .
- Validate with fluorogenic or chromogenic assays : For example, incorporate cleavage-sensitive fluorescent tags (e.g., EDANS/DABCYL) into synthetic peptide substrates to monitor real-time enzymatic activity .
Q. How can researchers address discrepancies in inhibitor efficacy data between HIV-1 and HIV-2 proteases?
Contradictions often arise due to:
- Structural polymorphisms : HIV-2 protease has natural polymorphisms (e.g., V32I, I54M) near the active site that reduce inhibitor binding affinity compared to HIV-1 .
- Assay variability : Standardize enzymatic assays (e.g., fluorescence resonance energy transfer) using recombinant proteases from clinically relevant strains. Include controls for enzyme dimerization stability, as HIV-2 protease is less stable in vitro .
- Cross-validate with cell-based assays : Compare enzymatic IC₅₀ values with antiviral EC₅₀ in infected T-cells to account for differences in cellular uptake or off-target effects .
Advanced Research Questions
Q. What structural insights inform the design of dual-target inhibitors for HIV-1 and HIV-2 proteases?
Key considerations include:
- Active-site flexibility : Despite ~40% sequence divergence, the catalytic Asp-Thr-Gly motif and substrate envelope are conserved. Inhibitors designed within this envelope (e.g., substrate-based hydroxyethylene isosteres) show cross-reactivity .
- Resistance-prone regions : Avoid targeting residues prone to mutation (e.g., HIV-2’s I54M polymorphism) by prioritizing interactions with highly conserved residues (e.g., Asp25, Gly27) .
- Use X-ray crystallography : Co-crystallize inhibitors with both proteases to identify binding discrepancies. For example, darunavir shows weaker binding to HIV-2 due to altered van der Waals contacts in the S2 pocket .
Q. How do HIV-2 proteases develop resistance to protease inhibitors (PIs) despite structural conservation?
HIV-2 exhibits intrinsic and acquired resistance through:
- Pre-existing polymorphisms : Natural variants (e.g., I82F in HIV-2) reduce PI binding without requiring new mutations, unlike HIV-1 .
- Dimer instability : HIV-2 protease’s lower dimerization stability allows partial activity even under inhibitor pressure, enabling viral replication .
- Limited clinical data : Monitor patients for mutations in both protease and Gag cleavage sites, as Gag mutations (e.g., A431V) can compensate for protease inhibitor efficacy .
Q. What methodologies enable high-resolution analysis of protease-substrate interactions under drug pressure?
Advanced approaches include:
- Cryo-EM and molecular dynamics (MD) : Resolve conformational changes in protease-substrate complexes during inhibitor binding. MD simulations reveal how mutations (e.g., HIV-2’s V32I) alter substrate-envelope flexibility .
- Deep mutational scanning : Profile all possible protease mutations to predict resistance pathways and identify vulnerabilities .
- Phylogenetic analysis : Use tools like the Stanford HIV Drug Resistance Database ( ) to correlate protease mutations with clinical outcomes across subtypes .
Q. Methodological Resources
Q. Which databases provide reliable structural and clinical data for HIV protease research?
- HIV Protease Database ( ): Contains 3D structures of HIV-1, HIV-2, and SIV proteases complexed with substrates/inhibitors. Filter by strain, inhibitor type, or publication year to identify conserved binding motifs .
- Stanford HIV Drug Resistance Database ( ): Curates protease mutation profiles linked to PI resistance, critical for validating experimental findings .
Q. How can researchers optimize enzyme kinetics assays for HIV-2 protease given its lower stability?
- Stabilize dimerization : Add kosmotropic salts (e.g., sodium citrate) or polyethylene glycol to enhance HIV-2 protease stability during assays .
- Use high-throughput screening (HTS) : Implement fluorescence polarization assays with short peptide substrates (e.g., SQNYPIVQ) to measure activity across a range of pH and temperature conditions .
Q. Data Contradiction Analysis
Q. Why do some studies report divergent substrate cleavage efficiencies between HIV-1 and HIV-2 proteases?
Discrepancies arise from:
Properties
Molecular Formula |
C56H80N12O14 |
---|---|
Molecular Weight |
1145.3 g/mol |
IUPAC Name |
(2S)-2-[[(2S)-1-[(2S)-2-[[(2S,3S)-2-[[(2S)-1-[(2S)-2-[[(2S)-4-amino-2-[[(2S)-2-[[(2S,3R)-2-[[(2S)-2-aminopropanoyl]amino]-3-hydroxybutanoyl]amino]-4-methylpentanoyl]amino]-4-oxobutanoyl]amino]-3-phenylpropanoyl]pyrrolidine-2-carbonyl]amino]-3-methylpentanoyl]amino]-3-hydroxypropanoyl]pyrrolidine-2-carbonyl]amino]-3-(1H-indol-3-yl)propanoic acid |
InChI |
InChI=1S/C56H80N12O14/c1-7-30(4)45(52(77)64-41(28-69)55(80)68-22-13-19-42(68)50(75)63-40(56(81)82)25-34-27-59-36-18-12-11-17-35(34)36)65-51(76)43-20-14-21-67(43)54(79)39(24-33-15-9-8-10-16-33)62-49(74)38(26-44(58)71)60-48(73)37(23-29(2)3)61-53(78)46(32(6)70)66-47(72)31(5)57/h8-12,15-18,27,29-32,37-43,45-46,59,69-70H,7,13-14,19-26,28,57H2,1-6H3,(H2,58,71)(H,60,73)(H,61,78)(H,62,74)(H,63,75)(H,64,77)(H,65,76)(H,66,72)(H,81,82)/t30-,31-,32+,37-,38-,39-,40-,41-,42-,43-,45-,46-/m0/s1 |
InChI Key |
VWDDSWUEJQYLTP-SOXXPWFNSA-N |
Isomeric SMILES |
CC[C@H](C)[C@@H](C(=O)N[C@@H](CO)C(=O)N1CCC[C@H]1C(=O)N[C@@H](CC2=CNC3=CC=CC=C32)C(=O)O)NC(=O)[C@@H]4CCCN4C(=O)[C@H](CC5=CC=CC=C5)NC(=O)[C@H](CC(=O)N)NC(=O)[C@H](CC(C)C)NC(=O)[C@H]([C@@H](C)O)NC(=O)[C@H](C)N |
Canonical SMILES |
CCC(C)C(C(=O)NC(CO)C(=O)N1CCCC1C(=O)NC(CC2=CNC3=CC=CC=C32)C(=O)O)NC(=O)C4CCCN4C(=O)C(CC5=CC=CC=C5)NC(=O)C(CC(=O)N)NC(=O)C(CC(C)C)NC(=O)C(C(C)O)NC(=O)C(C)N |
Origin of Product |
United States |
Disclaimer and Information on In-Vitro Research Products
Please be aware that all articles and product information presented on BenchChem are intended solely for informational purposes. The products available for purchase on BenchChem are specifically designed for in-vitro studies, which are conducted outside of living organisms. In-vitro studies, derived from the Latin term "in glass," involve experiments performed in controlled laboratory settings using cells or tissues. It is important to note that these products are not categorized as medicines or drugs, and they have not received approval from the FDA for the prevention, treatment, or cure of any medical condition, ailment, or disease. We must emphasize that any form of bodily introduction of these products into humans or animals is strictly prohibited by law. It is essential to adhere to these guidelines to ensure compliance with legal and ethical standards in research and experimentation.