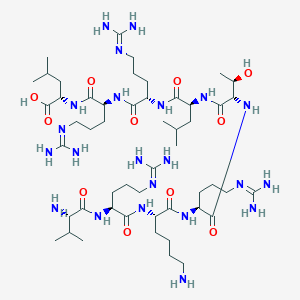
Protein Kinase C Substrate
- Click on QUICK INQUIRY to receive a quote from our team of experts.
- With the quality product at a COMPETITIVE price, you can focus more on your research.
Overview
Description
Protein Kinase C Substrate is a compound that plays a crucial role in the phosphorylation process mediated by Protein Kinase C enzymes. Protein Kinase C enzymes are a family of serine/threonine kinases involved in various cellular processes, including signal transduction, cell proliferation, and apoptosis . These enzymes phosphorylate substrates on serine or threonine residues, altering their biological activity and stabilizing specific conformational states .
Preparation Methods
Synthetic Routes and Reaction Conditions: The preparation of Protein Kinase C Substrate typically involves the synthesis of peptide sequences that mimic natural phosphorylation sites. These peptides are synthesized using solid-phase peptide synthesis, where amino acids are sequentially added to a growing peptide chain anchored to a solid support . The reaction conditions include the use of protecting groups to prevent unwanted side reactions and the use of coupling reagents to facilitate peptide bond formation .
Industrial Production Methods: Industrial production of this compound involves large-scale peptide synthesis using automated peptide synthesizers. These synthesizers can produce peptides with high purity and yield by optimizing reaction conditions and using high-quality reagents . The peptides are then purified using techniques such as high-performance liquid chromatography to remove any impurities .
Chemical Reactions Analysis
Types of Reactions: Protein Kinase C Substrate undergoes various chemical reactions, including phosphorylation, oxidation, and dephosphorylation . Phosphorylation is the primary reaction, where Protein Kinase C enzymes transfer a phosphate group from adenosine triphosphate to the substrate . Oxidation reactions involve the modification of cysteine residues by reactive oxygen species, altering the substrate’s activity .
Common Reagents and Conditions: Common reagents used in these reactions include adenosine triphosphate for phosphorylation and reactive oxygen species for oxidation . The reactions typically occur under physiological conditions, with specific cofactors such as calcium ions and diacylglycerol required for Protein Kinase C activation .
Major Products Formed: The major products formed from these reactions include phosphorylated substrates, which have altered biological activity and can participate in various cellular processes . Oxidized substrates may also form, which can affect the substrate’s function and stability .
Scientific Research Applications
Key Applications
-
Cancer Research
- PKC substrates are implicated in various cancers, making them targets for therapeutic intervention. For instance, studies have shown that different PKC isoforms (α, δ, ζ) interact with distinct substrates in breast cancer cells, influencing cell proliferation and survival pathways .
- Case Study : In a study involving MCF-10A cells, Cdc42 effector protein-4 was identified as a novel substrate for PKC isoforms, highlighting its potential role in cancer progression .
-
Neurobiology
- PKC substrates are critical in neuronal signaling and plasticity. The epsilon isoform of PKC (PKCε) has been shown to regulate synaptic transmission and is involved in neurodegenerative diseases.
- Case Study : Research has demonstrated that PKCε phosphorylates specific substrates that modulate synaptic strength, indicating its role in learning and memory processes .
-
Cardiovascular Research
- PKC substrates are involved in cardiac signaling pathways that regulate heart function. Alterations in PKC activity can lead to heart diseases.
- Case Study : Investigations into PKCε's role in cardiac myocytes revealed its involvement in hypertrophic signaling pathways, suggesting potential therapeutic targets for heart failure .
-
Drug Development
- Identifying specific PKC substrates aids in the development of selective inhibitors that can modulate kinase activity without affecting other kinases.
- Case Study : A substrate-mediated selection method was employed to discover small molecules that inhibit Src-dependent signaling pathways, demonstrating a novel approach to drug discovery targeting PKC-related pathways .
Table 1: Kinetic Parameters of Synthetic Peptides as Substrates for PKC
Peptide Sequence | Km (µM) | Vmax (nmol/min/mg) | Specificity |
---|---|---|---|
KRPSQRAKY | 13 | 2.5 | High |
Lys-Arg-Pro-Ser-Gln-Arg-Ala-Lys-Tyr | 20 | 1.8 | Moderate |
All-D Peptide | 50 | 0.5 | Low |
Note: Kinetic parameters were derived from Michaelis-Menten kinetics analysis .
Table 2: Identified Substrates of PKC Isoforms in Cancer Research
Substrate Name | Associated PKC Isoform | Role in Cancer |
---|---|---|
Cdc42 Effector Protein-4 | α, δ, ζ | Cell proliferation |
p53 | α | Tumor suppression |
Bad | ε | Apoptosis regulation |
Note: This table summarizes findings from various studies focusing on PKC substrates in cancer biology .
Mechanism of Action
The mechanism of action of Protein Kinase C Substrate involves its phosphorylation by Protein Kinase C enzymes. The enzymes recognize specific amino acid sequences on the substrate and transfer a phosphate group from adenosine triphosphate to serine or threonine residues . This phosphorylation event alters the substrate’s conformation and activity, enabling it to participate in various cellular processes . The molecular targets and pathways involved include signal transduction pathways mediated by diacylglycerol and calcium ions .
Comparison with Similar Compounds
Protein Kinase C Substrate can be compared with other kinase substrates, such as those for cyclin-dependent kinases and tyrosine kinases . While all these substrates undergo phosphorylation, this compound is unique in its requirement for specific cofactors such as calcium ions and diacylglycerol . Similar compounds include substrates for cyclin-dependent kinases, tyrosine kinases, and mitogen-activated protein kinases . These substrates differ in their recognition motifs and the specific kinases that phosphorylate them .
Biological Activity
Protein Kinase C (PKC) is a family of serine/threonine kinases that play critical roles in various cellular functions, including differentiation, proliferation, and apoptosis. The biological activity of PKC substrates is essential for understanding the signaling pathways mediated by PKC. This article provides a comprehensive overview of the biological activity of PKC substrates, focusing on their mechanisms, specificity, and implications in health and disease.
Overview of Protein Kinase C
PKC comprises a large family of enzymes with ten isoforms categorized into three groups based on their cofactor requirements: conventional (cPKCs), novel (nPKCs), and atypical (aPKCs). Each isoform exhibits distinct tissue distribution and regulatory mechanisms. The activation of cPKCs requires diacylglycerol (DAG), calcium ions, and phosphatidylserine, while nPKCs require only DAG and phosphatidylserine. Atypical PKCs do not depend on DAG or calcium for activation .
PKC substrates are primarily phosphorylated at serine and threonine residues. The phosphorylation process involves the binding of PKC to its substrate through specific recognition sequences. Research has shown that the substrate binding site is flexible, allowing for variations in substrate structure while maintaining phosphorylation capabilities .
Case Study: Pleckstrin
Pleckstrin is a well-studied PKC substrate involved in monocyte/macrophage signaling. It undergoes phosphorylation that induces conformational changes affecting its interaction with phosphoinositides. This interaction modulates intracellular calcium signaling and PKC activation .
Substrate Specificity
Substrate specificity is a critical aspect of PKC activity. Studies have demonstrated that the presence of certain amino acids can significantly influence the binding affinity and phosphorylation efficiency of substrates. For instance, the substitution of L-amino acids with D-amino acids in peptide substrates has been shown to affect kinetic parameters such as Km (Michaelis constant) and Vmax (maximum velocity) for phosphorylation reactions .
Table 1: Kinetic Parameters for PKC Substrates
Peptide Sequence | Km (µM) | Vmax (pmol/min) |
---|---|---|
KRPSQRAKY | 10 | 200 |
D-KRPSQRAKY | 25 | 150 |
L-KRPSQRAKY | 5 | 300 |
The data indicate that even minor modifications to peptide structure can lead to significant changes in enzymatic activity, highlighting the importance of substrate design in therapeutic applications.
Research Findings
Recent studies have identified various novel substrates for different PKC isoforms. For example, M2 pyruvate kinase has been recognized as a substrate for δPKC, demonstrating unique interactions not observed with other isoforms. This specificity suggests that targeting specific PKC pathways could provide therapeutic benefits in conditions like cancer and metabolic disorders .
Implications in Disease
The dysregulation of PKC activity and its substrates has been implicated in numerous diseases, including cancer, cardiovascular diseases, and neurodegenerative disorders. For instance, aberrant PKC signaling can lead to uncontrolled cell proliferation and survival, contributing to tumorigenesis . Understanding the specific roles of different PKC substrates may aid in developing targeted therapies.
Properties
Molecular Formula |
C51H100N22O11 |
---|---|
Molecular Weight |
1197.5 g/mol |
IUPAC Name |
(2S)-2-[[(2S)-2-[[(2S)-2-[[(2S)-2-[[(2S,3R)-2-[[(2S)-2-[[(2S)-6-amino-2-[[(2S)-2-[[(2S)-2-amino-3-methylbutanoyl]amino]-5-(diaminomethylideneamino)pentanoyl]amino]hexanoyl]amino]-5-(diaminomethylideneamino)pentanoyl]amino]-3-hydroxybutanoyl]amino]-4-methylpentanoyl]amino]-5-(diaminomethylideneamino)pentanoyl]amino]-5-(diaminomethylideneamino)pentanoyl]amino]-4-methylpentanoic acid |
InChI |
InChI=1S/C51H100N22O11/c1-26(2)24-35(44(80)69-31(15-10-20-62-48(54)55)40(76)67-32(16-11-21-63-49(56)57)42(78)72-36(47(83)84)25-27(3)4)71-46(82)38(29(7)74)73-43(79)34(18-13-23-65-51(60)61)68-39(75)30(14-8-9-19-52)66-41(77)33(17-12-22-64-50(58)59)70-45(81)37(53)28(5)6/h26-38,74H,8-25,52-53H2,1-7H3,(H,66,77)(H,67,76)(H,68,75)(H,69,80)(H,70,81)(H,71,82)(H,72,78)(H,73,79)(H,83,84)(H4,54,55,62)(H4,56,57,63)(H4,58,59,64)(H4,60,61,65)/t29-,30+,31+,32+,33+,34+,35+,36+,37+,38+/m1/s1 |
InChI Key |
PBWMKSKSLNNGHP-GWMUGDFHSA-N |
Isomeric SMILES |
C[C@H]([C@@H](C(=O)N[C@@H](CC(C)C)C(=O)N[C@@H](CCCN=C(N)N)C(=O)N[C@@H](CCCN=C(N)N)C(=O)N[C@@H](CC(C)C)C(=O)O)NC(=O)[C@H](CCCN=C(N)N)NC(=O)[C@H](CCCCN)NC(=O)[C@H](CCCN=C(N)N)NC(=O)[C@H](C(C)C)N)O |
Canonical SMILES |
CC(C)CC(C(=O)NC(CCCN=C(N)N)C(=O)NC(CCCN=C(N)N)C(=O)NC(CC(C)C)C(=O)O)NC(=O)C(C(C)O)NC(=O)C(CCCN=C(N)N)NC(=O)C(CCCCN)NC(=O)C(CCCN=C(N)N)NC(=O)C(C(C)C)N |
Origin of Product |
United States |
Disclaimer and Information on In-Vitro Research Products
Please be aware that all articles and product information presented on BenchChem are intended solely for informational purposes. The products available for purchase on BenchChem are specifically designed for in-vitro studies, which are conducted outside of living organisms. In-vitro studies, derived from the Latin term "in glass," involve experiments performed in controlled laboratory settings using cells or tissues. It is important to note that these products are not categorized as medicines or drugs, and they have not received approval from the FDA for the prevention, treatment, or cure of any medical condition, ailment, or disease. We must emphasize that any form of bodily introduction of these products into humans or animals is strictly prohibited by law. It is essential to adhere to these guidelines to ensure compliance with legal and ethical standards in research and experimentation.