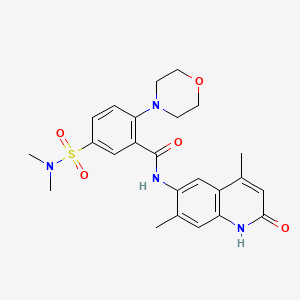
Bromodomain inhibitor-9
- Click on QUICK INQUIRY to receive a quote from our team of experts.
- With the quality product at a COMPETITIVE price, you can focus more on your research.
Overview
Description
Bromodomain inhibitor-9 (I-BRD9) is a selective small-molecule inhibitor targeting the bromodomain-containing protein 9 (BRD9), a member of the SWI/SNF chromatin remodeling complex. BRD9 plays a critical role in transcriptional regulation by recognizing acetylated lysine (KAc) residues on histones. Dysregulation of BRD9 is implicated in cancers, inflammation, and epigenetic disorders . I-BRD9 was developed to probe BRD9-specific biology, exhibiting high selectivity over other bromodomains, including BRD7 and BET family members. Its discovery involved structure-based optimization, leveraging crystallographic data to enhance binding affinity and specificity .
Preparation Methods
The synthesis of bromodomain inhibitor-9 involves several steps, including the formation of key intermediates and final coupling reactions. One common synthetic route starts with the preparation of a core scaffold, followed by functional group modifications to enhance selectivity and potency. Reaction conditions typically involve the use of organic solvents, catalysts, and controlled temperatures to achieve high yields and purity. Industrial production methods may include large-scale synthesis using automated reactors and purification techniques such as chromatography .
Chemical Reactions Analysis
Bromodomain inhibitor-9 undergoes various chemical reactions, including:
Oxidation: This reaction involves the addition of oxygen or the removal of hydrogen, often using reagents like hydrogen peroxide or potassium permanganate.
Reduction: This reaction involves the addition of hydrogen or the removal of oxygen, typically using reagents like sodium borohydride or lithium aluminum hydride.
Substitution: This reaction involves the replacement of one functional group with another, often using reagents like halogens or nucleophiles. Common reagents and conditions used in these reactions include organic solvents, acids, bases, and controlled temperatures.
Scientific Research Applications
Cancer Treatment
BRD9 has been identified as a promising target in various cancers, including acute myeloid leukemia (AML), hepatocellular carcinoma (HCC), and rhabdoid tumors. The inhibition of BRD9 has shown to:
- Reduce Cell Proliferation : Inhibition of BRD9 leads to decreased proliferation of cancer cells. For instance, studies have demonstrated that BRD9 inhibitors significantly suppress the growth of AML cells by disrupting enhancer-mediated MYC expression .
- Induce Apoptosis : Targeting BRD9 has been associated with increased apoptosis in tumor cells. In uterine fibroids, the use of BRD9 inhibitors resulted in enhanced apoptotic activity and reduced extracellular matrix deposition .
- Inhibit Metastasis : In HCC models, BRD9 knockdown curtailed cell migration and invasion, indicating its role in metastatic processes .
Epigenetic Therapy
BRD9's function as an epigenetic reader makes it a valuable target for epigenetic therapies aimed at reprogramming gene expression profiles in diseases such as cancer:
- Regulation of Oncogenes : BRD9 promotes the expression of oncogenes like TUFT1 through epigenetic mechanisms involving histone acetylation . Inhibiting BRD9 disrupts this process, providing a potential therapeutic avenue for cancers driven by such pathways.
- Combination Therapies : The synergistic effects of combining BRD9 inhibitors with other therapeutic agents have been explored. For example, combining BRD9 inhibitors with CDK inhibitors showed promise in reducing inflammation and preventing post-traumatic osteoarthritis development .
Case Studies
Mechanism of Action
Bromodomain inhibitor-9 exerts its effects by selectively binding to the acetyl-lysine recognition pocket of bromodomain-containing protein 9. This binding prevents the interaction of bromodomain-containing protein 9 with acetylated histones, thereby inhibiting its role in chromatin remodeling and transcriptional regulation. The molecular targets and pathways involved include the inhibition of gene expression, modulation of cell cycle progression, and suppression of oncogenic signaling pathways .
Comparison with Similar Compounds
Potency and Binding Affinity
I-BRD9 demonstrates nanomolar affinity for BRD9, with a dissociation constant (Kd) of 142 nM, as determined by isothermal titration calorimetry (ITC) . Compared to other BRD9 inhibitors:
- LP99 : A dual BRD7/9 inhibitor with moderate selectivity, showing a Kd of 909 nM for BRD9 and 3,400 nM for BRD7 .
- LP9918 : A BRD9-selective analog of LP99, with improved potency (Kd = 99 nM) and minimal BRD7 binding (ΔTm shift <1°C) .
- Bromosporine : A pan-bromodomain inhibitor with weaker BRD9 affinity (Kd >1 µM) and poor selectivity .
- GSK2801 : A BAZ2A/BRD9 inhibitor with comparable BRD9 affinity (Kd = 120 nM) but broader activity against BAZ2A, limiting its utility for BRD9-specific studies .
Table 1: Comparative Potency of BRD9 Inhibitors
Selectivity and Thermodynamic Profiling
I-BRD9 achieves selectivity through enthalpy-driven interactions, as revealed by ITC and differential scanning fluorimetry (DSF). Key structural features include:
- A triazolo[1,5-a]pyrazine scaffold forming hydrogen bonds with Asn100 and Tyr106 in the BRD9 KAc binding pocket.
- Hydrophobic interactions with Pro53 and Leu94, absent in BRD7 due to steric clashes with Val87 .
In contrast, LP99 and LP9918 use an indolizine core but differ in substituents; LP9918’s carbamate group enhances BRD9 selectivity by occupying a hydrophobic subpocket unique to BRD9 . Bromosporine lacks selectivity due to its rigid isoxazole ring, which indiscriminately binds conserved KAc sites .
Mechanistic Insights from Structural Studies
Crystal structures of BRD9 bound to I-BRD9 (1.5 Å resolution) highlight:
- A water-mediated hydrogen bond network stabilizing the inhibitor-protein interface.
- Conformational flexibility in the ZA loop of BRD9, accommodating I-BRD9’s methylpiperazine group .
Molecular dynamics simulations further reveal that I-BRD9’s selectivity arises from stronger van der Waals (vdW) interactions with BRD9’s Trp45 and weaker clashes with BRD7’s Val87 . Comparatively, GSK2801 adopts a distinct binding mode, engaging BAZ2A-specific residues like Glu1732, reducing its BRD9 specificity .
Therapeutic Potential and Limitations
- I-BRD9: Shows efficacy in AML and synovial sarcoma models by disrupting SWI/SNF-driven oncogenic transcription . However, its cell permeability is moderate, requiring optimization for in vivo use.
- LP9918: Exhibits superior pharmacokinetics (oral bioavailability = 65% in mice) and reduces tumor growth in BRD9-dependent xenografts .
- GSK2801 : Synergizes with BET inhibitors (e.g., JQ1) in triple-negative breast cancer, but off-target BAZ2A effects complicate mechanistic studies .
Table 2: Therapeutic Profiles of BRD9 Inhibitors
Biological Activity
Bromodomain inhibitors, particularly I-BRD9, have garnered significant attention in cancer research due to their ability to selectively inhibit bromodomain-containing proteins, which play crucial roles in chromatin remodeling and gene expression regulation. This article provides a comprehensive overview of the biological activity of I-BRD9, including its mechanisms of action, selectivity, and implications in cancer therapy.
Chemical Characteristics
Chemical Name: 5-Ethyl-4,5-dihydro-4-oxo-N-(tetrahydro-1,1-dioxido-2H-thiopyran-4-yl)-7-[3-(trifluoromethyl)phenyl]thieno[3,2-c]pyridine-2-carboximidamide
Purity: ≥98%
Selectivity: I-BRD9 exhibits over 70-fold selectivity for BRD9 compared to a panel of 34 other bromodomains and over 700-fold selectivity over the BET family .
I-BRD9 functions by binding to the bromodomain of BRD9, preventing its interaction with acetylated lysines on histones. This inhibition leads to the downregulation of several genes implicated in cancer progression, including CLEC1 , DUSP6 , FES , and SAMSN1 in Kasumi-1 cells . The selective inhibition is crucial as it minimizes off-target effects associated with less selective compounds.
Biological Activity and Efficacy
Research indicates that I-BRD9 effectively disrupts the function of SWI/SNF chromatin-remodeling complexes, which are often mutated in various cancers. The inhibition of BRD9 has been shown to:
- Induce Apoptosis: Inhibition leads to increased expression of pro-apoptotic factors such as BIM (BCL2L11), promoting tumor cell death through mitochondrial pathways .
- Suppress Tumor Growth: Studies have demonstrated that I-BRD9 can inhibit the proliferation of cancer cells by modulating key oncogenes like c-MYC and BCL2 .
- Alter Immune Microenvironment: By affecting signaling pathways such as STAT3, I-BRD9 enhances the presence of CD3+ and CD8+ lymphocytes, potentially improving anti-tumor immunity .
Case Studies and Clinical Implications
Several studies have explored the therapeutic potential of I-BRD9 in different cancer models:
- Acute Myeloid Leukemia (AML): A study highlighted that AML cells are dependent on BRD9 for survival. Inhibition using I-BRD9 resulted in significant reductions in cell viability and induced apoptosis in vitro .
- Ovarian Cancer Models: Research utilizing patient-derived xenograft (PDX) models showed that I-BRD9 effectively suppressed tumor growth by targeting specific oncogenic pathways, demonstrating its potential as a therapeutic agent against resistant ovarian cancer subtypes .
- Prostate Cancer: In preclinical models, I-BRD9 was shown to inhibit the interaction between BRD4 and androgen receptors, which is critical for tumor progression in prostate cancer .
Structure-Activity Relationship (SAR)
The selectivity and potency of I-BRD9 have been characterized through structure-activity relationship studies. These studies reveal that modifications to the chemical structure can significantly impact binding affinity and selectivity towards BRD9 over BRD7. For example:
Compound | Selectivity for BRD9 | Binding Affinity (Kd) |
---|---|---|
I-BRD9 | High | 7.3 nM |
BI-7273 | Low | 9 nM |
BI-9564 | Moderate | 12-fold selective |
These findings underscore the importance of chemical modifications in enhancing inhibitor selectivity while minimizing off-target interactions .
Q & A
Basic Research Questions
Q. What structural features of Bromodomain inhibitor-9 ensure selective targeting of BRD9 over homologous bromodomains like BRD7 or BET family members?
Selective inhibition of BRD9 requires exploiting structural differences in the ZA loop and hydrophobic binding pockets. For example, I-BRD9 achieves >200-fold selectivity over BRD7 by incorporating a methyl group that clashes with BRD7's Leu95, while maintaining interactions with BRD9's Leu131 and Tyr106 . Co-crystal structures reveal that hydrogen bonding with Asn100 and hydrophobic interactions with Tyr106/Leu131 are critical for BRD9 specificity .
Q. What experimental methods are used to validate the cellular activity of BRD9 inhibitors in epigenetic studies?
Cellular target engagement can be validated using RNA sequencing (RNA-seq) to identify BRD9-regulated genes (e.g., oncogenic or immune response pathways in Kasumi-1 cells) . Complementary assays include CETSA (Cellular Thermal Shift Assay) to confirm binding in live cells and chromatin immunoprecipitation (ChIP) to assess displacement of BRD9 from acetylated histone marks .
Q. How can fragment-based screening guide the discovery of novel BRD9 inhibitor scaffolds?
Fragment libraries screened via X-ray crystallography or surface plasmon resonance (SPR) identify low-affinity hits that bind conserved motifs (e.g., acetyl-lysine mimetics). For BRD9, fragments with a central aromatic ring and hydrogen-bonding groups (e.g., amides) are optimized using structure-activity relationship (SAR) studies. Shared interaction features (e.g., Asn140 in BRD4) can inform pan-bromodomain templates, while selectivity is achieved through fragment elongation into BRD9-specific pockets .
Q. What biochemical assays are standard for evaluating BRD9 inhibitor potency and selectivity?
Time-resolved fluorescence resonance energy transfer (TR-FRET) assays using recombinant BRD9 bromodomains and acetylated histone peptides (e.g., H3K14ac) measure IC50 values. Counter-screening against BET bromodomains (BRD2/3/4) and BRD7 ensures selectivity. Isothermal titration calorimetry (ITC) quantifies binding thermodynamics, while thermal shift assays (TSA) confirm stabilization of the BRD9-inhibitor complex .
Advanced Research Questions
Q. How can researchers resolve discrepancies between biochemical binding data and cellular efficacy for BRD9 inhibitors?
Contradictions may arise from off-target effects, cellular permeability, or epigenetic redundancy. Integrate molecular dynamics (MD) simulations to assess binding mode stability under physiological conditions . Free energy perturbation (FEP) calculations refine affinity predictions by modeling solvation and entropy changes . Validate using orthogonal cellular models (e.g., BRD9-knockout cells) to isolate phenotype-specific effects .
Q. What strategies mitigate off-target effects against BET bromodomains during BRD9 inhibitor optimization?
Structure-guided design focuses on residues divergent between BRD9 and BET proteins. For example, BRD9 lacks the conserved WPF shelf (Trp81 in BRD4), allowing incorporation of bulky substituents that clash with BET domains. Additionally, targeting the BRD9-specific "histidine gatekeeper" (His437) with charged groups improves selectivity .
Q. How do mutations in the BRD9 ligand-binding site impact inhibitor efficacy, and how can this be modeled computationally?
Mutations (e.g., Asn100Ala or Tyr106Phe) disrupt hydrogen bonding or hydrophobic packing. Use in silico saturation mutagenesis combined with MD simulations to predict resistance mechanisms. Interaction fingerprinting (e.g., PLIF) quantifies residue contribution scores, while alchemical free energy calculations (e.g., FEP) estimate ∆∆G changes upon mutation .
Q. What in vivo models are appropriate for assessing the therapeutic potential of BRD9 inhibitors in oncology?
Xenograft models using BRD9-dependent cancers (e.g., AML or neuroblastoma with MYCN amplification) are prioritized. Pharmacodynamic endpoints include histone acetylation levels (via LC-MS/MS) and BRD9 target gene suppression (e.g., MYC). For toxicity, monitor BET-dependent pathways (e.g., hematopoiesis) to ensure selectivity .
Q. How can computational methods accelerate the design of BRD9 inhibitors with improved brain permeability for neurological applications?
Machine learning models predict blood-brain barrier (BBB) penetration using descriptors like polar surface area (<90 Ų) and logP (2–5). Molecular docking against BRD9’s acetyl-lysine binding site prioritizes scaffolds with rigid, planar geometries. MD simulations assess stability in lipid bilayers to refine pharmacokinetic properties .
Q. What integrated approaches validate the epigenetic mechanism of BRD9 inhibitors in drug-resistant cancers?
Combine ChIP-seq to map BRD9 occupancy at drug-resistance loci (e.g., ABC transporters) with CRISPR interference (CRISPRi) to confirm gene regulation. RNA-seq profiles post-treatment transcriptomes, while ATAC-seq evaluates chromatin accessibility changes. Cross-reference with clinical datasets (e.g., TCGA) to identify BRD9-high patient cohorts likely to respond .
Methodological Notes
- Selectivity Optimization : Prioritize bromodomains with <70% sequence identity to BRD9 for counter-screening .
- Data Reproducibility : Use orthogonal assays (e.g., SPR + ITC) to confirm binding constants .
- Clinical Translation : Validate BRD9 dependency using patient-derived organoids (PDOs) before advancing to trials .
Properties
Molecular Formula |
C24H28N4O5S |
---|---|
Molecular Weight |
484.6 g/mol |
IUPAC Name |
N-(4,7-dimethyl-2-oxo-1H-quinolin-6-yl)-5-(dimethylsulfamoyl)-2-morpholin-4-ylbenzamide |
InChI |
InChI=1S/C24H28N4O5S/c1-15-12-23(29)25-21-11-16(2)20(14-18(15)21)26-24(30)19-13-17(34(31,32)27(3)4)5-6-22(19)28-7-9-33-10-8-28/h5-6,11-14H,7-10H2,1-4H3,(H,25,29)(H,26,30) |
InChI Key |
WVIPVRJNDZWQGP-UHFFFAOYSA-N |
Canonical SMILES |
CC1=CC2=C(C=C1NC(=O)C3=C(C=CC(=C3)S(=O)(=O)N(C)C)N4CCOCC4)C(=CC(=O)N2)C |
Origin of Product |
United States |
Disclaimer and Information on In-Vitro Research Products
Please be aware that all articles and product information presented on BenchChem are intended solely for informational purposes. The products available for purchase on BenchChem are specifically designed for in-vitro studies, which are conducted outside of living organisms. In-vitro studies, derived from the Latin term "in glass," involve experiments performed in controlled laboratory settings using cells or tissues. It is important to note that these products are not categorized as medicines or drugs, and they have not received approval from the FDA for the prevention, treatment, or cure of any medical condition, ailment, or disease. We must emphasize that any form of bodily introduction of these products into humans or animals is strictly prohibited by law. It is essential to adhere to these guidelines to ensure compliance with legal and ethical standards in research and experimentation.