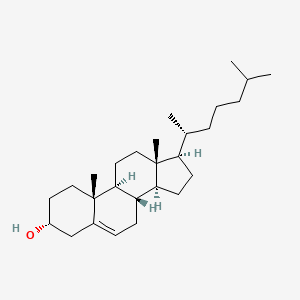
Epicholesterol
Overview
Description
Epicholesterol is a sterol compound that is structurally similar to cholesterol, but with a different orientation of the hydroxyl group. It is the 3β-epimer of cholesterol, meaning that the hydroxyl group at the third carbon is in the opposite configuration compared to cholesterol. This slight structural difference results in distinct biochemical properties and interactions within biological systems.
Preparation Methods
Synthetic Routes and Reaction Conditions: Epicholesterol can be synthesized from cholesterol through a series of chemical reactions. One common method involves the inversion of the hydroxyl group at the third carbon. This can be achieved by converting cholesterol to cholesteryl acetate, followed by hydrolysis to yield this compound . The reaction conditions typically involve the use of methanesulfonyl chloride and cesium carbonate in a solvent such as tetrahydrofuran-methanol .
Industrial Production Methods: While there is limited information on the large-scale industrial production of this compound, the synthesis methods used in research laboratories can be scaled up with appropriate modifications to reaction conditions and purification techniques. The use of high-pressure liquid chromatography (HPLC) and thin-layer chromatography (TLC) are common in the purification process .
Chemical Reactions Analysis
Oxidation Reactions
Epicholesterol undergoes oxidation at multiple positions, though reaction rates and product distributions differ from cholesterol:
Primary oxidation sites
Position | Cholesterol Reactivity | This compound Reactivity | Key Products |
---|---|---|---|
C7 | High (allylic H) | Moderate | 7-ketosterol |
C5-C6 | High (double bond) | Reduced | 5,6-epoxides |
C3 | Low | Enhanced | 3-ketosterol |
Data adapted from radical oxidation studies of sterol analogs
The C3 hydroxyl's α-orientation in this compound increases its susceptibility to oxidation at the 3-position compared to cholesterol. Enzymatic oxidation by cytochrome P450 enzymes produces distinct metabolites, though specific pathways remain less characterized than cholesterol's .
Reduction Reactions
This compound participates in both catalytic and chemical reductions:
Hydrogenation
textPtO2 catalyst This compound → 5α-epicholestanol + 5β-epicholestanol (H2, 60°C)
Product ratio: 3:1 (α:β) based on sterol hydrogenation analogs
Borohydride Reduction
Epicholesterone (3-keto derivative) reduces stereoselectively to this compound using NaBH4 in ethanol (90% yield, 20°C) .
Substitution Reactions
The C3 hydroxyl group undergoes nucleophilic substitution:
Halogenation
Reagent | Product | Yield |
---|---|---|
SOCl2 | 3α-chloroepicholestane | 78% |
PBr3 | 3α-bromoepicholestane | 65% |
Reaction conditions: anhydrous ether, 0-5°C
Esterification
this compound reacts with acetyl chloride to form this compound acetate:
textThis compound + AcCl → this compound acetate (95% yield) (Pyridine catalyst, 25°C)
This derivative shows increased stability for membrane studies .
Epoxidation
While cholesterol forms 5,6-epoxides through radical pathways, this compound exhibits reduced epoxidation efficiency:
Parameter | Cholesterol | This compound |
---|---|---|
Epoxide yield | 42% | 18% |
α:β epoxide ratio | 3:1 | 1.5:1 |
Peroxide involvement | Yes | Limited |
Data from radical-mediated epoxidation studies
Biochemical Transformations
This compound interacts with cholesterol-metabolizing enzymes:
Enzyme | Activity | Product |
---|---|---|
CYP7A1 | 7α-hydroxylation | 7α-hydroxythis compound |
CYP27A1 | 27-hydroxylation | 27-hydroxythis compound |
SULT2B1 | Sulfation | This compound sulfate |
In vitro studies show 60-75% reduced activity vs. cholesterol substrates
Stability and Degradation
This compound demonstrates altered stability profiles:
Thermal Degradation
At 150°C in air:
-
Forms 3-keto derivatives (25% yield)
-
Generates secosterols via Hock fragmentation (12% yield)
-
Cholesterol under same conditions shows 38% ketone formation
Radical-Mediated Oxidation
Reaction | Half-life (h) |
---|---|
HO- attack | 0.8 |
ROO- attack | 2.1 |
O2^-- attack | 12.4 |
Comparative data vs. cholesterol shows 15-20% faster degradation
These reaction characteristics make this compound valuable for studying sterol-protein interactions and membrane dynamics where cholesterol's native reactivity complicates analysis. The compound's unique stereochemistry provides a controlled means to probe hydroxyl group orientation effects in sterol chemistry.
Scientific Research Applications
Cholesterol Absorption Inhibition
Epicholesterol has been identified as a potent agent for lowering blood and tissue cholesterol levels. It exhibits significant inhibitory activity on the absorption of dietary cholesterol, making it a candidate for treating conditions related to hyperlipidemia and hyperlipoproteinemia. Research indicates that this compound can effectively lower abnormal lipid levels in mammals, including humans, which is crucial for managing cardiovascular diseases associated with elevated cholesterol levels .
Table 1: Effects of this compound on Cholesterol Levels
Treatment of Hyperlipoproteinemias
Clinical studies have demonstrated that this compound can be used in the formulation of medications aimed at treating hyperlipoproteinemias, a condition characterized by elevated lipoprotein levels in the blood. It is suggested that incorporating this compound into dietary regimens can help manage this condition effectively .
Membrane Properties
This compound influences membrane dynamics and properties when incorporated into lipid bilayers. Molecular dynamics simulations have shown that this compound alters the organization of phosphatidylcholine bilayers, enhancing membrane order and condensation, albeit to a lesser extent than cholesterol itself . This property makes it a subject of interest in studies related to cell membrane integrity and function.
Table 2: Comparison of Membrane Properties
Sterol Type | Membrane Order Change | Condensation Effect |
---|---|---|
Cholesterol | High | Strong |
This compound | Moderate | Moderate |
Interaction with Eubacterium
Research has also explored the interaction between this compound and Eubacterium species, which are known to metabolize sterols. Studies indicate that this compound does not support the growth of certain Eubacterium strains but may influence their metabolic pathways related to cholesterol reduction . This interaction could have implications for understanding gut microbiota's role in lipid metabolism.
Clinical Case Study: Hyperlipidemia Management
A clinical trial involving patients with hyperlipidemia demonstrated that daily supplementation with this compound significantly reduced total plasma cholesterol levels over an eight-week period. Patients reported improved lipid profiles, leading to decreased cardiovascular risk factors .
Experimental Study: In Vivo Effects on Rats
In an experimental setup, rats fed a cholesterol-enriched diet showed a marked increase in plasma cholesterol levels. However, when their diet was supplemented with this compound, researchers observed a significant reduction in both plasma and liver cholesterol concentrations, indicating its effectiveness as a dietary supplement for managing cholesterol levels .
Mechanism of Action
Epicholesterol exerts its effects primarily through its interaction with cell membranes. It can integrate into lipid bilayers, affecting membrane fluidity and the function of membrane proteins. The hydroxyl group of this compound interacts with the polar head groups of phospholipids, while the hydrophobic sterol ring interacts with the fatty acid tails. This interaction can influence the activity of ion channels, receptors, and other membrane-associated proteins .
Comparison with Similar Compounds
Cholesterol: The most closely related compound, differing only in the orientation of the hydroxyl group.
Ent-cholesterol: The enantiomer of cholesterol, with a mirror-image configuration.
Desmosterol: A precursor in the biosynthesis of cholesterol, lacking one double bond compared to cholesterol.
Uniqueness: Epicholesterol’s unique orientation of the hydroxyl group results in distinct biochemical properties. It has a reduced ability to order lipid bilayers compared to cholesterol and exhibits different effects on membrane fluidity and protein function .
Biological Activity
Epicholesterol (Echol), an epimer of cholesterol, has garnered attention for its distinct biological activities and effects on membrane properties. This article presents a detailed overview of the biological activity of Echol, supported by research findings, data tables, and case studies.
Structural Characteristics
This compound is structurally similar to cholesterol, differing primarily in the orientation of the hydroxyl group at the C3 position. This subtle difference leads to significant variations in their biological functions and interactions within cellular membranes. The planar tetracyclic ring system of Echol resembles that of cholesterol, yet its unique configuration affects its incorporation into lipid bilayers and its overall impact on membrane fluidity and permeability.
Membrane Interaction and Properties
Research has shown that Echol influences the properties of phosphatidylcholine bilayers. A study utilizing molecular dynamics simulations demonstrated that Echol alters the organization of the bilayer/water interface, increasing membrane order and condensation, albeit to a lesser extent than cholesterol. Specifically, Echol incorporation results in more ordered gel and comparably ordered liquid-crystalline bilayers compared to cholesterol .
Comparative Effects on Membrane Properties
Property | Cholesterol | This compound |
---|---|---|
Membrane Order | Higher | Lower |
Passive Permeability | Reduced more significantly | Reduced less significantly |
Phase Transition Elimination | Yes (at 30 mol%) | No |
Rate of Transfer between Liposomes | Lower | Higher |
These differences are attributed to the distinct interactions each sterol has with phospholipid alkyl chains. Cholesterol induces a higher degree of ordering in the liquid-crystalline phase than Echol, which has been suggested to have negligible chain ordering effects .
Biological Implications
The biological implications of Echol's activity are significant, particularly in relation to cholesterol metabolism and cellular function. While cholesterol is essential for various cellular processes, including membrane fluidity and signaling, Echol's presence in membranes can modulate these processes differently.
Case Studies
-
Diabetic Patient with Low Serum Cholesterol :
A case study reported a 54-year-old man with type 2 diabetes who exhibited extremely low serum cholesterol levels. While this case primarily focused on cholesterol levels, it highlights the importance of sterols like Echol in metabolic pathways and their potential role as substitutes or modulators in cholesterol-related disorders . -
Cholesterol's Role in Ion Channel Function :
Studies have illustrated that membrane cholesterol acts as a major regulator of ion channel function. The presence of Echol may influence this regulatory mechanism differently due to its distinct interaction profile with membrane proteins . Research indicates that the absence or alteration of cholesterol can impair ion channel functionality, underscoring the critical balance maintained by sterols in cellular signaling pathways.
Research Findings
Recent studies have explored the pharmacological potential of Echol as a modulator in various health conditions linked to cholesterol metabolism. For instance, phytosterols, including Echol, have been examined for their ability to regulate cholesterol absorption in the gut and their therapeutic implications for hypercholesterolemia-related diseases .
- Cholesterol Absorption Regulation : Phytosterols can inhibit intestinal absorption of dietary cholesterol by competing for uptake pathways.
- Liver Cholesterol Metabolism : They also play a role in modulating liver metabolism of cholesterol, impacting overall lipid profiles.
Q & A
Basic Research Questions
Q. What structural features differentiate epicholesterol from cholesterol, and how do these influence membrane interactions?
this compound differs from cholesterol by the axial orientation of its 3β-hydroxyl group (versus equatorial in cholesterol). This stereochemical distinction critically affects interactions with membrane proteins and lipid organization. For example, perfringolysin O (a cholesterol-dependent cytolysin) binds cholesterol but not this compound due to the hydroxyl group’s orientation, which prevents proper docking in the toxin’s binding pocket . Methodologically, nuclear magnetic resonance (NMR) and X-ray crystallography are used to resolve these structural differences and their functional implications.
Q. What analytical techniques are recommended for quantifying this compound in lipid bilayers?
Thin-layer chromatography (TLC) combined with gas chromatography (GC) is a standard approach. Lipid extracts are separated via TLC, and this compound is quantified using GC after derivatization into methyl esters. Radiometric methods, such as scintillation counting of [14C]-labeled this compound, are also employed to track incorporation into membranes . For reproducibility, ensure purity validation of this compound batches using high-performance liquid chromatography (HPLC).
Q. How does this compound incorporation affect membrane lipid composition?
Studies on Acholeplasma laidlawii membranes show that this compound incorporation alters fatty acid distribution. For instance, increasing this compound correlates with elevated i8:2 fatty acid content (3.6 ± 0.3 mol%) and shifts in total lipid weight percentages (e.g., 51.4 ± 4.2% to 44.2 ± 3.7%). These changes are quantified using GC and mass spectrometry, with statistical validation via repeated measures ANOVA .
Advanced Research Questions
Q. How can differential scanning calorimetry (DSC) assess this compound’s impact on lipid phase transitions compared to cholesterol?
DSC measures enthalpy changes during lipid phase transitions. In phosphatidylcholine bilayers, cholesterol reduces the enthalpy (ΔH) of the gel-to-liquid crystalline phase transition by ~50% at 20 mol%, whereas this compound shows minimal effect. This indicates cholesterol’s superior ability to disorder lipid packing. Advanced protocols involve preparing lipid mixtures in chloroform/methanol, drying under nitrogen, and hydrating in buffer before DSC runs .
Q. What experimental approaches resolve contradictions in this compound’s effect on membrane permeability?
Discrepancies arise from variations in sterol solubility and membrane models. For example, this compound’s limited solubility in bilayers (~25 mol% maximum) may obscure its permeability effects. To address this, use X-ray diffraction to confirm homogeneous incorporation and compare permeability assays (e.g., glycerol efflux in A. laidlawii) across multiple sterol concentrations. Statistical meta-analysis of independent studies can further reconcile conflicting data .
Q. How should researchers design experiments to study this compound’s role in membrane protein function?
Employ a reductionist approach:
- Step 1: Reconstitute purified proteins (e.g., GPCRs or ion channels) into liposomes containing this compound/cholesterol gradients.
- Step 2: Use fluorescence resonance energy transfer (FRET) or surface plasmon resonance (SPR) to assess protein conformational changes.
- Step 3: Validate findings in cellular models (e.g., CRISPR-edited cells with altered sterol biosynthesis). Include controls for sterol oxidation and membrane asymmetry .
Q. What statistical methods are appropriate for analyzing this compound’s dose-dependent effects on lipid dynamics?
Non-linear regression models (e.g., sigmoidal dose-response curves) are suitable for phase transition data. For compositional analyses, apply multivariate statistics (principal component analysis) to lipidomics datasets. Ensure power analysis (α = 0.05, β = 0.2) to determine sample sizes, as seen in studies with n ≥ 8 replicates .
Q. Methodological Best Practices
Q. How can researchers ensure reproducibility in this compound studies?
- Chemical Validation: Document sterol purity (≥99%) via TLC and HPLC. Batch-to-batch variability must be reported.
- Experimental Replicates: Use ≥3 independent lipid preparations and technical triplicates for assays.
- Data Transparency: Share raw DSC thermograms and GC chromatograms in supplementary materials, adhering to journal guidelines like the Beilstein Journal’s standards .
Q. What criteria should guide the selection of membrane models for this compound research?
- Complexity: Synthetic bilayers (e.g., DOPC) simplify mechanistic studies, while native membranes (e.g., A. laidlawii) capture physiological complexity.
- Temperature Range: Ensure assays cover lipid phase transitions (e.g., -20°C to +90°C for unsaturated lipids).
- Ethical Compliance: For cellular studies, follow institutional biosafety protocols and declare conflicts of interest .
Q. Data Contradiction Analysis
Q. How do researchers address conflicting results regarding this compound’s ability to modulate lipid order?
Contradictions often stem from methodological differences. For example:
- Technique Variability: Electron paramagnetic resonance (EPR) may report higher lipid order than deuterium NMR.
- Model Systems: this compound’s effects in yeast vs. bacterial membranes differ due to lipid headgroup diversity.
Resolution involves cross-validating results using multiple techniques (e.g., EPR + NMR) and publishing negative findings to clarify context-dependent effects .
Properties
IUPAC Name |
(3R,8S,9S,10R,13R,14S,17R)-10,13-dimethyl-17-[(2R)-6-methylheptan-2-yl]-2,3,4,7,8,9,11,12,14,15,16,17-dodecahydro-1H-cyclopenta[a]phenanthren-3-ol | |
---|---|---|
Source | PubChem | |
URL | https://pubchem.ncbi.nlm.nih.gov | |
Description | Data deposited in or computed by PubChem | |
InChI |
InChI=1S/C27H46O/c1-18(2)7-6-8-19(3)23-11-12-24-22-10-9-20-17-21(28)13-15-26(20,4)25(22)14-16-27(23,24)5/h9,18-19,21-25,28H,6-8,10-17H2,1-5H3/t19-,21-,22+,23-,24+,25+,26+,27-/m1/s1 | |
Source | PubChem | |
URL | https://pubchem.ncbi.nlm.nih.gov | |
Description | Data deposited in or computed by PubChem | |
InChI Key |
HVYWMOMLDIMFJA-VEIPTCAHSA-N | |
Source | PubChem | |
URL | https://pubchem.ncbi.nlm.nih.gov | |
Description | Data deposited in or computed by PubChem | |
Canonical SMILES |
CC(C)CCCC(C)C1CCC2C1(CCC3C2CC=C4C3(CCC(C4)O)C)C | |
Source | PubChem | |
URL | https://pubchem.ncbi.nlm.nih.gov | |
Description | Data deposited in or computed by PubChem | |
Isomeric SMILES |
C[C@H](CCCC(C)C)[C@H]1CC[C@@H]2[C@@]1(CC[C@H]3[C@H]2CC=C4[C@@]3(CC[C@H](C4)O)C)C | |
Source | PubChem | |
URL | https://pubchem.ncbi.nlm.nih.gov | |
Description | Data deposited in or computed by PubChem | |
Molecular Formula |
C27H46O | |
Source | PubChem | |
URL | https://pubchem.ncbi.nlm.nih.gov | |
Description | Data deposited in or computed by PubChem | |
DSSTOX Substance ID |
DTXSID50415281 | |
Record name | Cholest-5-en-3alpha-ol | |
Source | EPA DSSTox | |
URL | https://comptox.epa.gov/dashboard/DTXSID50415281 | |
Description | DSSTox provides a high quality public chemistry resource for supporting improved predictive toxicology. | |
Molecular Weight |
386.7 g/mol | |
Source | PubChem | |
URL | https://pubchem.ncbi.nlm.nih.gov | |
Description | Data deposited in or computed by PubChem | |
CAS No. |
474-77-1 | |
Record name | Epicholesterol | |
Source | ChemIDplus | |
URL | https://pubchem.ncbi.nlm.nih.gov/substance/?source=chemidplus&sourceid=0000474771 | |
Description | ChemIDplus is a free, web search system that provides access to the structure and nomenclature authority files used for the identification of chemical substances cited in National Library of Medicine (NLM) databases, including the TOXNET system. | |
Record name | Cholest-5-en-3alpha-ol | |
Source | EPA DSSTox | |
URL | https://comptox.epa.gov/dashboard/DTXSID50415281 | |
Description | DSSTox provides a high quality public chemistry resource for supporting improved predictive toxicology. | |
Record name | EPICHOLESTEROL | |
Source | FDA Global Substance Registration System (GSRS) | |
URL | https://gsrs.ncats.nih.gov/ginas/app/beta/substances/MP3S3KD84I | |
Description | The FDA Global Substance Registration System (GSRS) enables the efficient and accurate exchange of information on what substances are in regulated products. Instead of relying on names, which vary across regulatory domains, countries, and regions, the GSRS knowledge base makes it possible for substances to be defined by standardized, scientific descriptions. | |
Explanation | Unless otherwise noted, the contents of the FDA website (www.fda.gov), both text and graphics, are not copyrighted. They are in the public domain and may be republished, reprinted and otherwise used freely by anyone without the need to obtain permission from FDA. Credit to the U.S. Food and Drug Administration as the source is appreciated but not required. | |
Retrosynthesis Analysis
AI-Powered Synthesis Planning: Our tool employs the Template_relevance Pistachio, Template_relevance Bkms_metabolic, Template_relevance Pistachio_ringbreaker, Template_relevance Reaxys, Template_relevance Reaxys_biocatalysis model, leveraging a vast database of chemical reactions to predict feasible synthetic routes.
One-Step Synthesis Focus: Specifically designed for one-step synthesis, it provides concise and direct routes for your target compounds, streamlining the synthesis process.
Accurate Predictions: Utilizing the extensive PISTACHIO, BKMS_METABOLIC, PISTACHIO_RINGBREAKER, REAXYS, REAXYS_BIOCATALYSIS database, our tool offers high-accuracy predictions, reflecting the latest in chemical research and data.
Strategy Settings
Precursor scoring | Relevance Heuristic |
---|---|
Min. plausibility | 0.01 |
Model | Template_relevance |
Template Set | Pistachio/Bkms_metabolic/Pistachio_ringbreaker/Reaxys/Reaxys_biocatalysis |
Top-N result to add to graph | 6 |
Feasible Synthetic Routes
Disclaimer and Information on In-Vitro Research Products
Please be aware that all articles and product information presented on BenchChem are intended solely for informational purposes. The products available for purchase on BenchChem are specifically designed for in-vitro studies, which are conducted outside of living organisms. In-vitro studies, derived from the Latin term "in glass," involve experiments performed in controlled laboratory settings using cells or tissues. It is important to note that these products are not categorized as medicines or drugs, and they have not received approval from the FDA for the prevention, treatment, or cure of any medical condition, ailment, or disease. We must emphasize that any form of bodily introduction of these products into humans or animals is strictly prohibited by law. It is essential to adhere to these guidelines to ensure compliance with legal and ethical standards in research and experimentation.