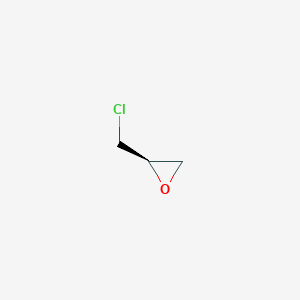
(R)-(-)-Epichlorohydrin
Overview
Description
(R)-(-)-Epichlorohydrin (C₃H₅ClO) is a chiral organochlorine epoxide first identified in 1848 during studies on glycerol and hydrogen chloride . It exists as a racemic mixture but can be enantiomerically resolved for applications requiring stereochemical precision. Its high reactivity stems from the epoxide ring and chlorine atom, enabling widespread use in synthesizing polymers (e.g., epoxy resins), adhesives, elastomers, and pharmaceuticals .
Preparation Methods
Enantioselective Hydrolysis Using Epoxide Hydrolases
Epoxide hydrolases (EHs) have emerged as biocatalysts for the kinetic resolution of racemic ECH. In a landmark study, recombinant epoxide hydrolase from Rhodotorula glutinis expressed in Pichia pastoris achieved enantioselective hydrolysis of racemic ECH in organic solvents . The reaction, conducted in n-dodecane with 2.5% (v/v) water, yielded (R)-ECH with 99% ee and 28.5% conversion within 60 minutes (Table 1). The use of organic solvents minimized spontaneous hydrolysis, enhancing enantioselectivity. This method’s scalability is limited by enzyme stability and the need for lyophilized whole-cell catalysts, but it offers an eco-friendly alternative to traditional chemical processes.
Table 1: Enzymatic Hydrolysis Parameters for (R)-ECH Synthesis
Substrate Concentration | Solvent System | Reaction Time | ee (%) | Yield (%) |
---|---|---|---|---|
20 mM | n-Dodecane | 60 min | 99 | 28.5 |
Chiral Catalysts and Kinetic Resolution
Kinetic resolution using chiral catalysts represents a cornerstone of asymmetric synthesis. A patented method employing dual-core Salen-Co(II) complexes with SnCl₄ or FeCl₃ achieved remarkable enantiocontrol . For instance, [(R,R)-SalenCo(II)]₂·SnCl₂ catalyzed the hydrolysis of racemic ECH in tetrahydrofuran (THF) at 25°C, yielding (R)-ECH with 99.6% ee and 40.2% yield in 2 hours (Table 2). The catalyst’s modular design allows tuning of steric and electronic effects, enabling precise control over reaction kinetics. However, catalyst synthesis involves multi-step procedures, and metal leaching remains a concern for large-scale applications.
Table 2: Performance of Salen-Co Catalysts in (R)-ECH Synthesis
Catalyst | Temperature (°C) | Time (h) | ee (%) | Yield (%) |
---|---|---|---|---|
[(R,R)-SalenCo(II)]₂·SnCl₂ | 25 | 2 | 99.6 | 40.2 |
[(S,S)-SalenCo(II)]₂·SnCl₄ | 30 | 20 | 99.0 | 38.3 |
Glycerol-Based Production: A Sustainable Route
The valorization of biodiesel byproduct glycerol into (R)-ECH has gained traction as a green chemistry initiative. A two-step process involving hydrochlorination and dehydrochlorination was optimized using hexanoic acid as a catalyst . In the first step, glycerol reacts with HCl at 383 K and 670 kPa, yielding 1,3-dichlorohydrin (1,3-DCH) with minimal trichloropropane byproducts. Subsequent ring closure with NaOH at 363 K produced ECH with 83% yield using Ti-MWW catalysts (Figure 1). The preferential formation of 1,3-DCH over 2,3-DCH (30–50:1 ratio) enhances cyclization efficiency, as 1,3-DCH reacts 300 times faster in basic conditions .
Figure 1: Glycerol-to-ECH Process Flow
-
Hydrochlorination : Glycerol + HCl → 1,3-DCH (hexanoic acid catalyst, 383 K, 670 kPa).
-
Cyclization : 1,3-DCH + NaOH → ECH (Ti-MWW catalyst, 363 K, 30 kPa).
Synthesis from Chiral Precursors: D-Mannitol
D-Mannitol, a naturally occurring sugar alcohol, serves as a chiral precursor for (R)-ECH synthesis. While detailed mechanistic studies are scarce, the process likely involves selective protection and oxidation steps to retain the desired (R)-configuration1. This route’s appeal lies in its reliance on renewable feedstocks, but scalability is hindered by multi-step synthesis and low overall yields.
Chemical Reactions Analysis
Types of Reactions: ®-(-)-Epichlorohydrin undergoes various chemical reactions, including:
Nucleophilic Substitution: The chlorine atom can be replaced by nucleophiles such as hydroxide ions, amines, and thiols.
Ring-Opening Reactions: The epoxide ring can be opened by nucleophiles, leading to the formation of diols, amino alcohols, and other derivatives.
Oxidation and Reduction: Epichlorohydrin can be oxidized to form glycidol or reduced to form 3-chloropropanol.
Common Reagents and Conditions:
Nucleophilic Substitution: Reagents such as sodium hydroxide, ammonia, and thiols are commonly used. The reactions are typically carried out at room temperature or slightly elevated temperatures.
Ring-Opening Reactions: Acids, bases, and various nucleophiles can be used to open the epoxide ring. The reactions are often conducted in solvents such as water, alcohols, or aprotic solvents.
Oxidation and Reduction: Oxidizing agents like hydrogen peroxide and reducing agents like lithium aluminum hydride are used under controlled conditions.
Major Products Formed:
Nucleophilic Substitution: Products include glycidol, amino alcohols, and thiol derivatives.
Ring-Opening Reactions: Products include diols, amino alcohols, and other functionalized compounds.
Oxidation and Reduction: Products include glycidol and 3-chloropropanol.
Scientific Research Applications
Production of Epoxy Resins
Epichlorohydrin is a key precursor in the synthesis of epoxy resins, which are widely used in coatings, adhesives, and composite materials. These resins are known for their excellent adhesion, chemical resistance, and mechanical properties, making them suitable for applications in the automotive and aerospace industries .
Application | Details |
---|---|
Coatings | Used for protective coatings on metal structures and flooring materials. |
Adhesives | Essential in formulating high-performance adhesives for various substrates. |
Composite Materials | Integral in manufacturing fiber-reinforced composites used in wind turbine blades and aircraft components. |
Synthesis of Glycerol Ethers
(R)-(-)-Epichlorohydrin serves as a building block for synthesizing glycerol ethers, which find applications in personal care products and pharmaceuticals. These ethers enhance the texture and stability of creams and lotions .
Intermediate in Chemical Synthesis
The compound acts as an important intermediate in the production of various chemicals, including pharmaceuticals and agrochemicals. Its versatility allows for the creation of diverse compounds tailored for specific applications .
Manufacture of Flame Retardants
It is utilized in producing flame retardants that improve the safety of materials used in construction and electronics, providing enhanced protection against fire hazards .
Research in Polymer Chemistry
In academic and industrial research settings, this compound is employed to study polymerization processes, aiding scientists in developing new materials with improved properties for applications such as packaging and insulation .
Case Study 1: Epoxy Resin Development
A notable case study involves the use of this compound in developing high-performance epoxy resins for aerospace applications. Researchers demonstrated that incorporating this compound into resin formulations significantly improved thermal stability and mechanical strength compared to traditional epoxy systems.
Case Study 2: Glycerol Ether Synthesis
In pharmaceutical research, this compound was successfully used to synthesize glycerol ethers that serve as excipients in drug formulations. These ethers were shown to enhance drug solubility and stability, leading to improved bioavailability.
Safety Considerations
While this compound has numerous beneficial applications, it is classified as a probable carcinogen by several health agencies. Prolonged exposure can lead to serious health risks, including lung irritation and increased cancer risk . Therefore, safety protocols must be strictly adhered to during its handling and application.
Mechanism of Action
The mechanism of action of ®-(-)-Epichlorohydrin involves its reactivity as an epoxide. The strained three-membered ring is highly reactive and can undergo nucleophilic attack, leading to ring-opening reactions. The chlorine atom also makes it susceptible to nucleophilic substitution reactions. These properties make it a versatile intermediate in various chemical transformations.
Comparison with Similar Compounds
Key Properties :
- Molecular weight: 92.52 g/mol
- Boiling point: 117.9°C
- Density: 1.18 g/cm³
- Enantiomeric purity: Up to 99% ee via biocatalytic methods .
Structural and Functional Analogues
Ethylene Oxide (C₂H₄O)
- Structure : Lacks the chlorine atom present in epichlorohydrin.
- Reactivity : Less electrophilic due to the absence of chlorine, limiting its utility in nucleophilic substitutions.
- Applications : Primarily used in sterilization and polyether production.
- Safety: Ethylene oxide is a known carcinogen and mutagen, whereas epichlorohydrin’s toxicity is less pronounced but still requires careful handling .
Glycidol (C₃H₆O₂)
- Structure : Contains a hydroxyl group instead of chlorine.
- Synthesis : More expensive to produce enantiopure forms; (S)-glycidol costs 16–20× more than (R)-epichlorohydrin .
- Applications : Used in β-blockers (e.g., metoprolol) but requires costlier resolution methods compared to epichlorohydrin’s biocatalytic routes .
Propylene Oxide (C₃H₆O)
- Structure : Methyl group replaces chlorine in epichlorohydrin.
- Reactivity : Lower polarity reduces suitability for crosslinking reactions.
- Applications : Production of polyurethanes and surfactants.
Market and Production
Biological Activity
(R)-(-)-Epichlorohydrin, a chiral epoxide compound, is recognized for its significant biological activity, particularly as an alkylating agent. This article explores its biological effects, mechanisms of action, and relevant case studies, supported by data tables and research findings.
This compound is a bifunctional alkylating agent that can form covalent bonds with nucleophilic sites in DNA and proteins. The compound's reactivity stems from its epoxide and chloride functionalities, which enable it to interact with various biological molecules. It is known to induce DNA interstrand cross-linking , a critical mechanism contributing to its cytotoxicity.
Key Mechanisms:
- DNA Cross-Linking : this compound forms interstrand cross-links primarily at deoxyguanosine residues, which can lead to DNA strand breaks and subsequent cellular apoptosis .
- Cytotoxicity : Studies indicate that this compound exhibits greater cytotoxicity than its enantiomer (S)-epichlorohydrin, with an IC50 value of approximately 2.2 mM compared to 3.9 mM for (S)-epichlorohydrin .
- Protein Cross-Linking : Besides DNA, this compound can also cross-link proteins, further contributing to its biological effects .
Cytotoxicity and Mutagenicity
The cytotoxic effects of this compound have been studied extensively in various cell lines. The compound's ability to induce DNA damage correlates strongly with its mutagenic potential.
- Cytotoxicity Studies : In experiments involving chicken erythroprogenitor cells, this compound demonstrated a dose-dependent increase in cytotoxicity .
- Mutagenicity : The compound has been classified as probably carcinogenic to humans (Group 2A) by the International Agency for Research on Cancer (IARC), based on evidence from animal studies showing increased tumor incidence following exposure .
Case Studies
Several studies have focused on the biological activity of this compound in different contexts:
-
Animal Studies : A study conducted on Wistar rats revealed a dose-dependent increase in forestomach lesions upon administration of epichlorohydrin. At higher doses (10 mg/kg bw), significant hyperplasia and carcinoma were observed .
Dose (mg/kg bw) Hyperplasia Papilloma Carcinoma Control 5/50 1/50 6/49 2 24/40 6/49 35/49 10 6/49 4/49 24/39 - Metabolic Studies : Research has shown that the metabolism of this compound involves conjugation with glutathione and hydration by epoxide hydrolase, leading to the formation of metabolites that may contribute to its toxic effects .
Toxicology and Safety Considerations
Exposure to this compound poses several health risks. Occupational exposure limits have been established due to its potential toxicity and carcinogenicity. The compound is known to cause respiratory irritation and has been associated with liver and kidney damage in animal studies .
Q & A
Basic Research Questions
Q. What are the standard synthetic routes for (R)-(-)-epichlorohydrin, and how do reaction conditions influence enantiomeric purity?
this compound is synthesized via two primary methods:
- Chlorohydrin Process : Propylene reacts with chlorine and water to form 1,2-dichloropropane, followed by dehydrochlorination using bases (e.g., NaOH) under controlled temperatures (0–50°C) .
- Glycerol Chlorination : A newer method uses glycerol (a biodiesel byproduct) reacted with HCl gas and catalysts (e.g., carboxylic acids) to produce 1,3-dichlorohydrin, which is then cyclized to epichlorohydrin . Enantiomeric purity depends on reaction kinetics, catalyst choice, and separation techniques (e.g., chiral chromatography). Industrial processes often yield racemic mixtures, requiring resolution steps for the (R)-enantiomer .
Q. What safety protocols are critical when handling this compound in laboratory settings?
- Personal Protective Equipment (PPE) : Gloves, goggles, and lab coats must be worn to avoid skin/eye contact, as the compound is a severe irritant and suspected carcinogen .
- Ventilation : Use fume hoods to minimize inhalation of vapors, which can cause pulmonary edema .
- Waste Disposal : Collect waste in sealed containers for professional hazardous waste treatment to prevent environmental contamination .
Q. How does this compound’s reactivity differ from its (S)-(+)-enantiomer in nucleophilic substitution reactions?
Both enantiomers undergo nucleophilic substitution (e.g., with amines or thiols), but their stereochemistry influences reaction pathways. For example, the (R)-enantiomer is preferred in synthesizing chiral pharmaceuticals like rivaroxaban, where configuration affects drug activity . Kinetic studies show slight differences in reaction rates due to steric effects in chiral environments .
Advanced Research Questions
Q. What enzymatic strategies enable enantioselective synthesis or resolution of this compound?
- Halohydrin Dehalogenases (HHDHs) : Enzymes like HheC catalyze the nitrite-mediated ring-opening of racemic epichlorohydrin, resolving this compound with >99% enantiomeric excess (ee) at 41% conversion .
- Epoxide Hydrolases : Coupled with HHDHs in two-phase systems, these enzymes enhance ee by selectively hydrolyzing the (S)-enantiomer .
- Biocatalytic Oxidation : Chloroperoxidase from Caldariomyces fumago enantioselectively oxidizes 3-chloropropene to this compound .
Q. How can trace-level quantification of this compound be achieved in pharmaceutical intermediates?
- Gas Chromatography-Headspace (GC-HS) : Validated methods with a detection limit of 50 ppm (for rivaroxaban synthesis) use DB-624 columns (30 m × 0.32 mm × 1.8 µm) and FID detectors. Parameters: split ratio 10:1, injector 200°C, detector 250°C, and oven ramp from 40°C (5 min) to 240°C (10°C/min) .
- LC-MS/MS : For sub-ppm detection, electrospray ionization in negative mode with MRM transitions (m/z 92.5 → 57.1) is employed .
Q. What experimental design principles optimize this compound’s use in polymer synthesis (e.g., β-cyclodextrin crosslinking)?
A factorial design study identified key variables for β-cyclodextrin/epichlorohydrin polymerization:
Q. How do data contradictions arise in toxicity studies of this compound, and how can they be resolved?
Discrepancies in carcinogenicity data (e.g., rodent vs. human studies) stem from metabolic differences. Rodents show higher susceptibility due to glutathione depletion pathways. Researchers must contextualize findings using:
- In vitro models : Human liver microsomes to assess metabolic activation.
- Dose-response analysis : Linear vs. threshold models for risk extrapolation .
- Comparative genomics : Identify conserved detoxification pathways across species .
Q. Methodological Tables
Table 1. Key Reaction Conditions for this compound Synthesis
Method | Catalyst | Temp. Range | Yield (%) | Purity (ee) | Reference |
---|---|---|---|---|---|
Chlorohydrin Process | NaOH | 0–50°C | 85–90 | Racemic | |
Glycerol Chlorination | Acetic Acid | 80–120°C | 70–75 | Racemic | |
Enzymatic Resolution | HheC + Nitrite | 25°C | 41 | >99% (R) |
Table 2. Analytical Parameters for GC-HS Quantification
Parameter | Value |
---|---|
Column | DB-624 (30 m × 0.32 mm × 1.8 µm) |
Detection Limit | 50 ppm |
Linearity Range | 50–200 ppm (R² > 0.999) |
Recovery Rate | 98–102% |
Properties
IUPAC Name |
(2R)-2-(chloromethyl)oxirane | |
---|---|---|
Source | PubChem | |
URL | https://pubchem.ncbi.nlm.nih.gov | |
Description | Data deposited in or computed by PubChem | |
InChI |
InChI=1S/C3H5ClO/c4-1-3-2-5-3/h3H,1-2H2/t3-/m0/s1 | |
Source | PubChem | |
URL | https://pubchem.ncbi.nlm.nih.gov | |
Description | Data deposited in or computed by PubChem | |
InChI Key |
BRLQWZUYTZBJKN-VKHMYHEASA-N | |
Source | PubChem | |
URL | https://pubchem.ncbi.nlm.nih.gov | |
Description | Data deposited in or computed by PubChem | |
Canonical SMILES |
C1C(O1)CCl | |
Source | PubChem | |
URL | https://pubchem.ncbi.nlm.nih.gov | |
Description | Data deposited in or computed by PubChem | |
Isomeric SMILES |
C1[C@@H](O1)CCl | |
Source | PubChem | |
URL | https://pubchem.ncbi.nlm.nih.gov | |
Description | Data deposited in or computed by PubChem | |
Molecular Formula |
C3H5ClO | |
Source | PubChem | |
URL | https://pubchem.ncbi.nlm.nih.gov | |
Description | Data deposited in or computed by PubChem | |
DSSTOX Substance ID |
DTXSID501014417 | |
Record name | (-)-Epichlorohydrin | |
Source | EPA DSSTox | |
URL | https://comptox.epa.gov/dashboard/DTXSID501014417 | |
Description | DSSTox provides a high quality public chemistry resource for supporting improved predictive toxicology. | |
Molecular Weight |
92.52 g/mol | |
Source | PubChem | |
URL | https://pubchem.ncbi.nlm.nih.gov | |
Description | Data deposited in or computed by PubChem | |
CAS No. |
51594-55-9 | |
Record name | (-)-Epichlorohydrin | |
Source | CAS Common Chemistry | |
URL | https://commonchemistry.cas.org/detail?cas_rn=51594-55-9 | |
Description | CAS Common Chemistry is an open community resource for accessing chemical information. Nearly 500,000 chemical substances from CAS REGISTRY cover areas of community interest, including common and frequently regulated chemicals, and those relevant to high school and undergraduate chemistry classes. This chemical information, curated by our expert scientists, is provided in alignment with our mission as a division of the American Chemical Society. | |
Explanation | The data from CAS Common Chemistry is provided under a CC-BY-NC 4.0 license, unless otherwise stated. | |
Record name | Epichlorohydrin, (-)- | |
Source | ChemIDplus | |
URL | https://pubchem.ncbi.nlm.nih.gov/substance/?source=chemidplus&sourceid=0051594559 | |
Description | ChemIDplus is a free, web search system that provides access to the structure and nomenclature authority files used for the identification of chemical substances cited in National Library of Medicine (NLM) databases, including the TOXNET system. | |
Record name | (-)-Epichlorohydrin | |
Source | EPA DSSTox | |
URL | https://comptox.epa.gov/dashboard/DTXSID501014417 | |
Description | DSSTox provides a high quality public chemistry resource for supporting improved predictive toxicology. | |
Record name | (R)-1-chloro-2,3-epoxypropane | |
Source | European Chemicals Agency (ECHA) | |
URL | https://echa.europa.eu/substance-information/-/substanceinfo/100.102.280 | |
Description | The European Chemicals Agency (ECHA) is an agency of the European Union which is the driving force among regulatory authorities in implementing the EU's groundbreaking chemicals legislation for the benefit of human health and the environment as well as for innovation and competitiveness. | |
Explanation | Use of the information, documents and data from the ECHA website is subject to the terms and conditions of this Legal Notice, and subject to other binding limitations provided for under applicable law, the information, documents and data made available on the ECHA website may be reproduced, distributed and/or used, totally or in part, for non-commercial purposes provided that ECHA is acknowledged as the source: "Source: European Chemicals Agency, http://echa.europa.eu/". Such acknowledgement must be included in each copy of the material. ECHA permits and encourages organisations and individuals to create links to the ECHA website under the following cumulative conditions: Links can only be made to webpages that provide a link to the Legal Notice page. | |
Record name | (2R)-(-)-3-Chloro-1,2-propenoxide | |
Source | European Chemicals Agency (ECHA) | |
URL | https://echa.europa.eu/information-on-chemicals | |
Description | The European Chemicals Agency (ECHA) is an agency of the European Union which is the driving force among regulatory authorities in implementing the EU's groundbreaking chemicals legislation for the benefit of human health and the environment as well as for innovation and competitiveness. | |
Explanation | Use of the information, documents and data from the ECHA website is subject to the terms and conditions of this Legal Notice, and subject to other binding limitations provided for under applicable law, the information, documents and data made available on the ECHA website may be reproduced, distributed and/or used, totally or in part, for non-commercial purposes provided that ECHA is acknowledged as the source: "Source: European Chemicals Agency, http://echa.europa.eu/". Such acknowledgement must be included in each copy of the material. ECHA permits and encourages organisations and individuals to create links to the ECHA website under the following cumulative conditions: Links can only be made to webpages that provide a link to the Legal Notice page. | |
Record name | EPICHLOROHYDRIN, (-)- | |
Source | FDA Global Substance Registration System (GSRS) | |
URL | https://gsrs.ncats.nih.gov/ginas/app/beta/substances/VEC01H609I | |
Description | The FDA Global Substance Registration System (GSRS) enables the efficient and accurate exchange of information on what substances are in regulated products. Instead of relying on names, which vary across regulatory domains, countries, and regions, the GSRS knowledge base makes it possible for substances to be defined by standardized, scientific descriptions. | |
Explanation | Unless otherwise noted, the contents of the FDA website (www.fda.gov), both text and graphics, are not copyrighted. They are in the public domain and may be republished, reprinted and otherwise used freely by anyone without the need to obtain permission from FDA. Credit to the U.S. Food and Drug Administration as the source is appreciated but not required. | |
Synthesis routes and methods I
Procedure details
Synthesis routes and methods II
Procedure details
Synthesis routes and methods III
Procedure details
Synthesis routes and methods IV
Procedure details
Retrosynthesis Analysis
AI-Powered Synthesis Planning: Our tool employs the Template_relevance Pistachio, Template_relevance Bkms_metabolic, Template_relevance Pistachio_ringbreaker, Template_relevance Reaxys, Template_relevance Reaxys_biocatalysis model, leveraging a vast database of chemical reactions to predict feasible synthetic routes.
One-Step Synthesis Focus: Specifically designed for one-step synthesis, it provides concise and direct routes for your target compounds, streamlining the synthesis process.
Accurate Predictions: Utilizing the extensive PISTACHIO, BKMS_METABOLIC, PISTACHIO_RINGBREAKER, REAXYS, REAXYS_BIOCATALYSIS database, our tool offers high-accuracy predictions, reflecting the latest in chemical research and data.
Strategy Settings
Precursor scoring | Relevance Heuristic |
---|---|
Min. plausibility | 0.01 |
Model | Template_relevance |
Template Set | Pistachio/Bkms_metabolic/Pistachio_ringbreaker/Reaxys/Reaxys_biocatalysis |
Top-N result to add to graph | 6 |
Feasible Synthetic Routes
Disclaimer and Information on In-Vitro Research Products
Please be aware that all articles and product information presented on BenchChem are intended solely for informational purposes. The products available for purchase on BenchChem are specifically designed for in-vitro studies, which are conducted outside of living organisms. In-vitro studies, derived from the Latin term "in glass," involve experiments performed in controlled laboratory settings using cells or tissues. It is important to note that these products are not categorized as medicines or drugs, and they have not received approval from the FDA for the prevention, treatment, or cure of any medical condition, ailment, or disease. We must emphasize that any form of bodily introduction of these products into humans or animals is strictly prohibited by law. It is essential to adhere to these guidelines to ensure compliance with legal and ethical standards in research and experimentation.