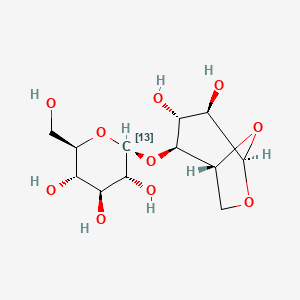
Cellobiosan-13C
- Click on QUICK INQUIRY to receive a quote from our team of experts.
- With the quality product at a COMPETITIVE price, you can focus more on your research.
Overview
Description
Cellobiosan-13C is a stable isotopologue of cellobiosan, a dehydrated derivative of cellobiose (a β-1,4-linked glucose dimer). The 13C labeling at specific positions enhances its utility in metabolic tracing, nuclear magnetic resonance (NMR) spectroscopy, and isotopic dilution studies. Unlike unlabeled cellobiosan, the incorporation of 13C allows researchers to track biochemical pathways with high precision, particularly in lignin degradation studies or carbohydrate metabolism .
Key properties of this compound include:
- Molecular formula: C₁₂H₂₂O₁₁ (with one or more 13C substitutions).
- Molecular weight: ~344.3 g/mol (varies slightly depending on 13C substitution sites).
- Isotopic purity: Typically ≥99% 13C enrichment.
- Solubility: Highly soluble in polar solvents (e.g., water, DMSO) due to hydroxyl-rich structure.
Its synthesis often involves acid-catalyzed pyrolysis of 13C-labeled cellulose, followed by chromatographic purification to isolate the dehydrated product .
Preparation Methods
Synthetic Routes and Reaction Conditions
Cellobiosan-13C is typically synthesized through the pyrolysis of cellulose that has been enriched with the carbon-13 isotope. The process involves heating the cellulose in the absence of oxygen, leading to the breakdown of the polymer into smaller anhydrosugars, including cellobiosan. The reaction conditions, such as temperature and heating rate, are carefully controlled to optimize the yield of this compound.
Industrial Production Methods
Industrial production of this compound follows similar principles but on a larger scale. The cellulose feedstock is first enriched with carbon-13, often through the use of carbon-13-labeled glucose during the growth of the cellulose-producing plants. The enriched cellulose is then subjected to pyrolysis in large reactors, and the resulting this compound is purified through various separation techniques, such as chromatography.
Chemical Reactions Analysis
Pyrolysis Reactions
Pyrolysis is a thermal decomposition process that occurs in the absence of oxygen. Recent studies have shown that the fast pyrolysis of Cellobiosan-13C leads to various products through fragmentation pathways. The primary findings include:
-
Fragmentation Pathways : The reducing end of cellobiose is particularly reactive, leading to the formation of smaller carbohydrate fragments and aromatic compounds. Research indicated that certain products arise entirely from the fragmentation of the reducing end while leaving the non-reducing end intact .
-
Product Analysis : Mass spectrometry coupled with pyrolysis revealed several products, including levoglucosan and other oligomers. Quantum chemical calculations were employed to elucidate low-energy pathways for these reactions, providing insights into the underlying mechanisms .
Hydrolysis Reactions
Hydrolysis involves the breakdown of compounds by reaction with water. For this compound, hydrolysis can be catalyzed by acids or enzymes:
-
Enzymatic Hydrolysis : Specific enzymes such as cellulases act on this compound to yield glucose units. Studies have shown that the presence of carbon-13 enhances NMR sensitivity, allowing for detailed tracking of reaction progress and product formation .
-
Acid Hydrolysis : Acidic conditions can also facilitate hydrolysis, yielding glucose and other oligosaccharides. The reaction kinetics can be monitored using NMR spectroscopy to observe changes in chemical shifts corresponding to different carbon environments .
Electrophilic Substitution Reactions
This compound can undergo electrophilic aromatic substitution due to its structural characteristics:
-
Mechanism Insights : In electrophilic aromatic substitution reactions involving derivatives of cellobiose, the introduction of electrophiles can lead to significant changes in molecular symmetry and NMR signal patterns. For instance, the addition of carboxyl groups alters the symmetry observed in NMR spectra, indicating successful substitution .
Nuclear Magnetic Resonance Spectroscopy
Nuclear magnetic resonance spectroscopy is pivotal in studying this compound due to its ability to provide detailed information about molecular structure:
-
Chemical Shift Analysis : The 13C NMR spectra reveal distinct peaks corresponding to different carbon environments within this compound. For example, shifts in the C4 region can indicate conformational changes or interactions with solvents .
Mass Spectrometry
Mass spectrometry complements NMR by providing information about molecular weight and fragmentation patterns:
-
Product Identification : Fast pyrolysis coupled with mass spectrometry has been used to identify various products resulting from the thermal degradation of this compound, enhancing understanding of its reactivity under different conditions .
Chemical Shift Assignments for this compound
Carbon Position | Chemical Shift (ppm) | Comments |
---|---|---|
C1 | 107.2 | Typical for anomeric carbon |
C2 | 72.9 | Reflects glycosidic linkage |
C3 | 73.5 | Similar environment as C2 |
C4 | 87.7 | Sensitive to conformation |
C5 | 75.0 | Stable under various conditions |
C6 | 62.5 | Lower shift due to hydroxyl group |
Pyrolysis Product Distribution
Product | Yield (%) | Formation Mechanism |
---|---|---|
Levoglucosan | 40 | Fragmentation of reducing end |
Aromatic Compounds | 30 | Secondary reactions during pyrolysis |
Oligomers | 20 | Partial degradation |
Unreacted this compound | 10 | Non-reactive under conditions tested |
Scientific Research Applications
Chemistry
Cellobiosan-13C is primarily used as a tracer in NMR spectroscopy. This application allows researchers to study:
- Molecular Structure : The carbon-13 label enhances the ability to determine the structure of complex molecules.
- Dynamics of Reactions : It helps in understanding reaction mechanisms and pathways in organic chemistry.
Biology
In biological research, this compound plays a crucial role in metabolic studies:
- Metabolic Pathways : It is employed to trace carbohydrate metabolism in various organisms, providing insights into how different species utilize carbohydrates.
- Microbial Studies : Specific soil microbes can metabolize cellobiosan as their sole carbon source, making it essential for studying microbial ecology and carbon cycling.
Medicine
This compound has potential applications in the medical field:
- Diagnostic Tools : It can assist in developing diagnostic methods for metabolic disorders by tracing metabolic pathways.
- Therapeutic Agents : Understanding its metabolism may lead to novel therapeutic approaches targeting specific metabolic pathways.
Industrial Applications
The compound also finds utility in industrial processes:
- Biofuel Production : this compound is significant in biofuel research, aiding in the understanding of biomass conversion processes.
- Bioplastics : It contributes to studies on bioplastics production, helping to optimize processes for sustainable materials.
Similar Compounds
Compound | Description | Applications |
---|---|---|
Levoglucosan | An anhydrosugar from cellulose pyrolysis | Similar tracer applications |
Cellobiose | A disaccharide derived from cellulose | Used in biochemical studies |
Glucose-13C | Labeled glucose used in metabolic studies | Tracing metabolic pathways |
Uniqueness of this compound
This compound is unique due to its specific structure and the presence of the carbon-13 isotope. This specificity enhances its utility in NMR spectroscopy and other analytical techniques, allowing for detailed information about molecular structures and dynamics.
This compound plays a significant role in the carbon cycle and is utilized by various microorganisms. Recent studies have identified several bacterial and fungal species capable of metabolizing cellobiosan:
Microbial Utilization
A pivotal study isolated six soil organisms capable of using cellobiosan as their sole carbon source:
Isolate Name | Closest Match (NCBI) | Growth on Cellobiosan | Growth on Levoglucosan |
---|---|---|---|
Sphingobacterium multivorum | 97% similarity | Yes | Yes |
Acinetobacter oleivorans JC3-1 | 98% similarity | Yes | Yes |
Enterobacter sp SJZ-6 | 99% similarity | Yes | Yes |
Microbacterium spp FXJ8.207 | 97% similarity | Yes | Yes |
Microbacterium spp FXJ8.203 | 97% similarity | Yes | Yes |
Cryptococcus sp | 95% similarity | Yes | No |
These findings indicate that cellobiosan is a more effective carbon source for these organisms compared to levoglucosan, highlighting its ecological significance.
Case Study 1: Microbial Metabolism
In a study published by PLOS ONE, researchers isolated six soil microbes that could utilize cellobiosan effectively. They demonstrated that these microbes showed significant growth when using cellobiosan compared to levoglucosan, indicating its potential as a superior carbon source for microbial metabolism .
Case Study 2: NMR Studies
An investigation into glucose and cellobiose metabolism using NMR spectroscopy revealed insights into how these compounds are processed by cellulolytic bacteria. The study highlighted how labeled compounds like this compound can provide detailed information about metabolic pathways .
Mechanism of Action
The mechanism of action of cellobiosan-13C involves its incorporation into metabolic pathways where it acts as a substrate for various enzymes. The carbon-13 label allows researchers to track the molecule through different biochemical processes, providing insights into the molecular targets and pathways involved. For example, in microbial metabolism, this compound is broken down by specific enzymes, and the labeled carbon atoms can be traced through the metabolic network to study the flow of carbon in the system.
Comparison with Similar Compounds
Cellobiosan-13C shares functional and structural similarities with other isotopically labeled carbohydrates and dehydrated sugars. Below is a detailed comparison based on physicochemical properties, synthesis methods, and applications.
Table 1: Physicochemical Properties
Key Differentiators
Isotopic Utility: this compound is uniquely suited for studying β-1,4 glycosidic bond cleavage in lignocellulosic biomass due to its dimeric structure and 13C labeling . In contrast, Glucose-13C₆ is preferred for monosaccharide-level metabolic flux analysis .
Synthesis Complexity: this compound requires controlled pyrolysis of 13C-labeled cellulose, a process sensitive to temperature and acid catalysts . Levoglucosan-13C, a monomeric analog, is synthesized via simpler anhydrous saccharide decomposition .
Solubility and Stability :
- This compound exhibits lower aqueous solubility (38.4 mg/ml) compared to Glucose-13C₆ (91 g/100 ml) but higher thermal stability than maltosan due to its dehydrated structure .
Cost and Accessibility: 13C-labeled disaccharides like this compound are significantly more expensive (≥$500/g) than monomeric analogs (e.g., Glucose-13C₆ at \sim$200/g) due to complex purification requirements .
Biological Activity
Cellobiosan-13C, a labeled form of cellobiosan, is a polysaccharide derived from cellulose, primarily consisting of two glucose units linked by a β-1,4-glycosidic bond. This compound has garnered attention due to its potential biological applications, particularly in microbial metabolism and biofuel production. This article provides an in-depth analysis of its biological activity, supported by relevant data tables and research findings.
Overview of Biological Activity
This compound plays a significant role in the carbon cycle and is utilized by various microorganisms. Its biological activity is primarily characterized by its degradation by specific soil microbes, which can metabolize it as a carbon source. Recent studies have identified several bacterial and fungal species capable of utilizing cellobiosan, contributing to our understanding of its ecological significance.
Microbial Utilization
A pivotal study investigated the microbial utilization of cellobiosan, identifying six soil isolates capable of using it as their sole carbon source. The isolates included:
-
Bacterial Isolates :
- Sphingobacterium multivorum
- Acinetobacter oleivorans JC3-1
- Enterobacter sp SJZ-6
- Microbacterium spp. FXJ8.207 and FXJ8.203
-
Fungal Isolate :
- Cryptococcus sp.
These isolates demonstrated significant growth on cellobiosan compared to levoglucosan, indicating that cellobiosan is a more effective carbon source for these organisms (Table 1) .
Isolate Name | Closest Match (NCBI) | Growth on Cellobiosan | Growth on Levoglucosan |
---|---|---|---|
Sphingobacterium multivorum | 97% similarity | Yes | Yes |
Acinetobacter oleivorans JC3-1 | 98% similarity | Yes | Yes |
Enterobacter sp SJZ-6 | 99% similarity | Yes | Yes |
Microbacterium spp FXJ8.207 | 97% similarity | Yes | Yes |
Microbacterium spp FXJ8.203 | 97% similarity | Yes | Yes |
Cryptococcus sp | 95% similarity | Yes | No |
Case Study 1: Soil Microbial Diversity
In a study focusing on soil microbial diversity, researchers isolated organisms from Iowa soil samples capable of metabolizing cellobiosan. The phylogenetic analysis revealed that these isolates are distinct from previously described anhydrosugar-utilizing microbes, suggesting a broader ecological role for cellobiosan in soil environments .
Case Study 2: Enzymatic Pathways
Another significant aspect of research on cellobiosan involves understanding the enzymatic pathways that facilitate its degradation. The identification of enzymes involved in the breakdown of cellobiosan could lead to advancements in biofuel production technologies. For instance, enzymes such as endoglucanases and exoglucanases play crucial roles in the hydrolysis of polysaccharides into fermentable sugars .
Research Findings
Recent findings indicate that the biological activity of cellobiosan is not only limited to microbial utilization but also extends to potential applications in biotechnological processes:
- Biofuel Production : Cellobiosan can serve as a substrate for microbial fermentation processes aimed at producing biofuels. The metabolic pathways identified in various microbes suggest that optimizing these pathways could enhance biofuel yield from biomass sources .
- Carbon Cycling : The ability of certain microorganisms to utilize cellobiosan contributes to carbon cycling in ecosystems, highlighting its ecological importance .
Q & A
Q. What are the standard analytical techniques for characterizing Cellobiosan-13C purity and isotopic enrichment?
Basic
To confirm purity and isotopic enrichment, researchers should employ:
- Nuclear Magnetic Resonance (NMR) : Use 13C-NMR at 125 MHz or higher to resolve labeled carbons, comparing chemical shifts with unlabeled cellobiosan. Integrate peak areas to quantify isotopic enrichment .
- Mass Spectrometry (MS) : High-resolution MS (e.g., ESI-TOF) in negative ion mode to detect isotopic patterns. Calculate 13C incorporation via mass difference (e.g., +1 Da per 13C atom) .
- Chromatography : Pair HPLC with refractive index detection to assess purity, ensuring retention times match unlabeled standards.
Q. How should researchers design experiments to ensure accurate quantification of this compound in metabolic flux studies?
Basic
- Internal Standards : Co-inject 2H-labeled analogs to correct for matrix effects .
- Calibration Curves : Prepare standards spanning physiological concentrations (e.g., 0.1–100 µM) in biological matrices (e.g., cell lysates) to validate linearity .
- Replicate Sampling : Collect triplicate samples at each time point to account for biological variability .
Q. What strategies resolve contradictory NMR spectral data when using this compound in complex biological matrices?
Advanced
- 2D NMR Techniques : Apply 1H-13C HSQC to resolve overlapping signals in crowded spectra. Compare with spiked pure standards to confirm assignments .
- Paramagnetic Relaxation Agents : Add chelated gadolinium to suppress background signals from macromolecules .
- Statistical Validation : Use principal component analysis (PCA) to distinguish artifacts from true isotopic enrichment patterns .
Q. How to validate the isotopic stability of this compound under varying physiological conditions?
Advanced
- Accelerated Stability Studies : Incubate this compound at 37°C in buffers (pH 4–9) for 72 hours. Monitor degradation via LC-MS and compare 13C retention to controls .
- Enzymatic Challenge : Expose to β-glucosidase and quantify unlabeled glucose release via enzymatic assays .
- Isotope Dilution MS : Spike stable isotope internal standards to track exchange reactions .
Q. What are the critical parameters for optimizing this compound synthesis to achieve >98% isotopic incorporation?
Basic
- Precursor Selection : Use 13C-glucose with >99% isotopic purity as the starting material .
- Reaction Monitoring : Track intermediates via TLC or inline IR spectroscopy to optimize reaction times .
- Purification : Employ size-exclusion chromatography to remove unreacted monomers, followed by lyophilization .
Q. How to address discrepancies between in vivo tracer kinetics and in vitro enzymatic assays using this compound?
Advanced
- Compartmental Modeling : Integrate in vivo tracer data with Michaelis-Menten parameters from in vitro assays to identify transport limitations .
- Metabolite Pool Size Correction : Normalize flux rates to intracellular ATP/ADP ratios to account for energy-dependent uptake .
- Knockout Controls : Use gene-edited cell lines (e.g., lacking specific transporters) to isolate uptake mechanisms .
Q. What controls are essential when using this compound in carbohydrate metabolism studies?
Basic
- Unlabeled Controls : Include parallel experiments with unlabeled cellobiosan to distinguish isotopic effects .
- Blank Matrices : Analyze cell-free media to identify background signals in MS/NMR .
- Enzyme-Inactivated Samples : Heat-denature enzymes to confirm tracer stability .
Q. What computational models best integrate this compound tracing data with genome-scale metabolic reconstructions?
Advanced
- Flux Balance Analysis (FBA) : Constrain models with 13C labeling patterns to predict pathway fluxes .
- Isotopic Non-Stationary Metabolic Flux Analysis (INST-MFA) : Use transient labeling data to resolve dynamic flux changes .
- Machine Learning : Train neural networks on multi-omics datasets to predict tracer incorporation hotspots .
Properties
Molecular Formula |
C12H20O10 |
---|---|
Molecular Weight |
325.27 g/mol |
IUPAC Name |
(2S,3R,4S,5S,6R)-2-[[(1R,2S,3R,4R,5R)-3,4-dihydroxy-6,8-dioxabicyclo[3.2.1]octan-2-yl]oxy]-6-(hydroxymethyl)(213C)oxane-3,4,5-triol |
InChI |
InChI=1S/C12H20O10/c13-1-3-5(14)6(15)8(17)12(20-3)22-10-4-2-19-11(21-4)9(18)7(10)16/h3-18H,1-2H2/t3-,4-,5-,6+,7-,8-,9-,10-,11-,12+/m1/s1/i12+1 |
InChI Key |
LTYZUJSCZCPGHH-WDQZVOSTSA-N |
Isomeric SMILES |
C1[C@@H]2[C@H]([C@@H]([C@H]([C@H](O1)O2)O)O)O[13C@H]3[C@@H]([C@H]([C@@H]([C@H](O3)CO)O)O)O |
Canonical SMILES |
C1C2C(C(C(C(O1)O2)O)O)OC3C(C(C(C(O3)CO)O)O)O |
Origin of Product |
United States |
Disclaimer and Information on In-Vitro Research Products
Please be aware that all articles and product information presented on BenchChem are intended solely for informational purposes. The products available for purchase on BenchChem are specifically designed for in-vitro studies, which are conducted outside of living organisms. In-vitro studies, derived from the Latin term "in glass," involve experiments performed in controlled laboratory settings using cells or tissues. It is important to note that these products are not categorized as medicines or drugs, and they have not received approval from the FDA for the prevention, treatment, or cure of any medical condition, ailment, or disease. We must emphasize that any form of bodily introduction of these products into humans or animals is strictly prohibited by law. It is essential to adhere to these guidelines to ensure compliance with legal and ethical standards in research and experimentation.