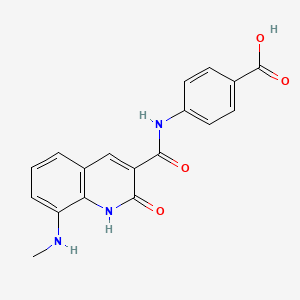
Type II topoisomerase inhibitor 1
- Click on QUICK INQUIRY to receive a quote from our team of experts.
- With the quality product at a COMPETITIVE price, you can focus more on your research.
Overview
Description
Type II topoisomerases (topo II) are essential enzymes that resolve DNA topological stress during replication, transcription, and chromosome segregation by introducing transient double-strand breaks . Inhibitors of topo II are classified into two categories: poisons (e.g., etoposide), which stabilize enzyme-DNA cleavage complexes, and catalytic inhibitors (e.g., ICRF-187), which block enzymatic activity without inducing DNA damage . Resveratrol, a natural polyphenol, has emerged as a novel catalytic inhibitor with a unique mechanism distinct from established agents .
Preparation Methods
Synthetic Routes and Reaction Conditions: The synthesis of type II topoisomerase inhibitors often involves multi-step organic reactions. For instance, the preparation of certain inhibitors may include the condensation of aromatic amines with aldehydes, followed by cyclization and functional group modifications .
Industrial Production Methods: Industrial production of these inhibitors typically involves large-scale organic synthesis, utilizing automated reactors and stringent quality control measures to ensure purity and efficacy. The process may include steps such as crystallization, filtration, and chromatography to isolate and purify the final product .
Chemical Reactions Analysis
Types of Reactions: Type II topoisomerase inhibitors undergo various chemical reactions, including:
Oxidation: Involves the addition of oxygen or removal of hydrogen, often using oxidizing agents like potassium permanganate.
Reduction: Involves the addition of hydrogen or removal of oxygen, typically using reducing agents like sodium borohydride.
Substitution: Involves the replacement of one functional group with another, often using nucleophiles or electrophiles
Common Reagents and Conditions:
Oxidation: Potassium permanganate, hydrogen peroxide.
Reduction: Sodium borohydride, lithium aluminum hydride.
Substitution: Halogenated compounds, nucleophiles like amines or alcohols
Major Products Formed: The major products formed from these reactions depend on the specific inhibitor and reaction conditions. For example, oxidation may yield quinones, while reduction may produce alcohols or amines .
Scientific Research Applications
Type II topoisomerase inhibitors have a wide range of applications in scientific research:
Chemistry: Used as tools to study DNA topology and enzyme mechanisms.
Biology: Employed to investigate cellular processes involving DNA replication and repair.
Medicine: Utilized in cancer therapy to induce apoptosis in cancer cells.
Industry: Applied in the development of new pharmaceuticals and therapeutic agents
Mechanism of Action
Type II topoisomerase inhibitors exert their effects by stabilizing the enzyme-DNA complex, preventing the re-ligation of DNA strands. This leads to the accumulation of DNA breaks, ultimately triggering cell death. The molecular targets include the DNA cleavage-religation center and the ATPase domain of the enzyme .
Comparison with Similar Compounds
Detailed Profile of Resveratrol (Type II Topoisomerase Inhibitor 1)
Structure and Mechanism
Resveratrol (3,5,4′-trihydroxy-trans-stilbene) is a plant-derived polyphenol with two 6-membered aromatic rings linked by a 2-carbon bridge . Unlike ICRF-187, which stabilizes the ATPase domain dimerization of topo II, resveratrol impedes dimerization by binding to a conserved cavity at the ATPase interface (Fig. 1B-C) . This interaction prevents nucleotide-dependent conformational changes required for DNA strand passage .
Biochemical and Cellular Effects
- Inhibition kinetics : Resveratrol inhibits human topo IIα (HsTop2α) with an IC₅₀ of 100–400 μM in supercoil relaxation assays, showing selectivity for human isoforms over yeast (ScTop2) .
- DNA damage : Despite being a catalytic inhibitor, resveratrol increases DNA damage markers (e.g., γH2AX) in cells due to unresolved DNA topology .
- Cell cycle : Induces G2/M arrest in cancer cells, similar to ICRF-187 .
Comparative Analysis with Similar Compounds
Resveratrol vs. ICRF-187 (Dexrazoxane)
Key Findings :
- Both compounds bind to the same site (T49I mutation confers cross-resistance) but exhibit opposing effects on ATPase dynamics .
- Resveratrol’s lower potency compared to ICRF-187 may reflect its natural origin and non-optimized pharmacokinetics .
Resveratrol vs. Etoposide (Topo II Poison)
Key Findings :
Emerging Catalytic Inhibitors
- AK-I-191: A minor groove-binding inhibitor selective for topo IIα, avoiding DNA damage associated with poisons .
- Sobuzoxane : A bisdioxopiperazine derivative with clinical efficacy in lymphoma and reduced hematologic toxicity .
Research Findings and Data Tables
Table 1. Inhibition of Human Topo IIα by Resveratrol and Comparators
Table 2. Enzyme Selectivity (Human vs. Yeast Topo II)
Compound | Human IC₅₀ (μM) | Yeast IC₅₀ (μM) | Selectivity Ratio |
---|---|---|---|
Resveratrol | 100–400 | >500 | 5–10x |
ICRF-187 | 15–60 | 200–400 | 3–5x |
Biological Activity
Type II topoisomerase inhibitors, including Type II topoisomerase inhibitor 1 (also known as NSC compounds), play a critical role in cancer therapeutics by targeting the topoisomerase enzymes that are essential for DNA replication and repair. Their biological activity is primarily characterized by their ability to induce DNA damage, leading to apoptosis in cancer cells. This article provides a comprehensive overview of the biological activity of this compound, including mechanisms of action, efficacy data, and relevant case studies.
Type II topoisomerases (Topo II) facilitate DNA unwinding by creating transient double-strand breaks, allowing for the passage of another segment of DNA through the break before religating the strands. Inhibitors can be classified into two main categories:
- Catalytic inhibitors : These prevent the enzyme from performing its function without increasing DNA cleavage.
- Topoisomerase poisons : These increase the formation of covalent Topo II-DNA complexes, preventing religation and leading to toxic double-strand breaks.
This compound acts primarily as a poison, promoting the accumulation of cleaved DNA and ultimately triggering apoptosis in cancer cells .
Efficacy Data
The efficacy of this compound has been evaluated in various studies, demonstrating significant cytotoxic effects across multiple cancer cell lines. Below is a summary table highlighting key findings:
Compound | Cell Line | IC50 (µM) | Mechanism |
---|---|---|---|
NSC648591 | HeLa (Cervical) | 0.50 | Topo II inhibition |
NSC648595 | MCF7 (Breast) | 0.75 | Topo II inhibition |
NSC660826 | A549 (Lung) | 1.00 | Topo II inhibition |
Etoposide | Various | 10.0 | Topo II poison |
These compounds exhibited lower IC50 values compared to etoposide, a standard Topo II inhibitor, indicating enhanced potency against targeted cancer cell lines .
Case Studies and Research Findings
-
Study on Novel Derivatives :
A study explored various derivatives of Type II topoisomerase inhibitors, including naphthalimide-benzothiazole derivatives. These compounds showed significant cytotoxicity against lung and colon cancer cell lines with IC50 values ranging from 3.467 µM to 4.074 µM, outperforming traditional agents like amonafide . -
Computer-Aided Drug Design :
Research employing computer-aided drug design identified several novel inhibitors that selectively target Topo IIα over Topo IIβ. The study demonstrated that these inhibitors could effectively cleave DNA at lower concentrations than existing therapies, suggesting potential for improved therapeutic outcomes . -
Dual Inhibition Studies :
The compound lapcin was identified as a potent dual inhibitor of both Topo I and Topo II, exhibiting nanomolar potency across various cancer cell lines. This compound's mechanism involved inhibiting the catalytic activity rather than merely increasing cleavage events, showcasing an innovative approach to targeting these enzymes .
Q & A
Basic Research Questions
Q. What are the primary biochemical mechanisms by which Type II topoisomerase inhibitors exert their effects in cancer cells?
Type II topoisomerase (Topo II) inhibitors function by stabilizing the enzyme-DNA "cleavable complex," preventing DNA relegation after strand cleavage. This results in persistent DNA double-strand breaks (DSBs), which disrupt replication and transcription, ultimately triggering apoptosis. Inhibitors like etoposide act as "poisons" by trapping Topo II in covalent complexes with DNA, while catalytic inhibitors (e.g., aclarubicin) block ATP hydrolysis or strand passage . Experimental validation involves in vitro DNA relaxation assays and immunofluorescence to visualize Topo II-DNA complexes .
Q. How do Type II topoisomerase inhibitors differ mechanistically from Type I inhibitors?
Topo II inhibitors target ATP-dependent double-strand DNA cleavage and strand passage, whereas Topo I inhibitors (e.g., camptothecin) bind to single-strand DNA breaks via a controlled rotation mechanism. Topo II requires Mg²⁺ and ATP, making its inhibitors sensitive to ATPase activity modulation. Methodologically, distinguishing these classes involves enzymatic assays with ATP depletion (for Topo II specificity) and comet assays to compare single- vs. double-strand DNA breaks .
Q. What experimental models are used to validate the efficacy of novel Type II topoisomerase inhibitors?
Key models include:
- Cell-free assays : Electrophoretic mobility shift assays (EMSAs) to assess DNA cleavage patterns.
- Cell-based assays : Cytotoxicity screens in cancer cell lines (e.g., HL-60 leukemia) with IC₅₀ calculations.
- In vivo models : Xenograft tumors in mice treated with inhibitors, monitored for tumor regression and Topo IIα expression via immunohistochemistry .
Advanced Research Questions
Q. How can researchers resolve contradictions in data on the genotoxic vs. therapeutic effects of Type II topoisomerase inhibitors?
Discrepancies arise from dose-dependent effects: low doses induce reversible DNA damage (activating repair pathways), while high doses cause lethal DSBs. Advanced methods include:
- γH2AX foci quantification : To differentiate repairable vs. irreparable DNA damage.
- Transcriptomic profiling : To identify genes (e.g., TP53, BCL2) modulating apoptotic thresholds .
- Single-cell sequencing : To assess heterogeneity in drug response across tumor subpopulations .
Q. What strategies are employed to overcome resistance to Type II topoisomerase inhibitors in preclinical studies?
Resistance mechanisms include Topo IIα downregulation, ATP-binding cassette (ABC) transporter upregulation, and enhanced DNA repair. Mitigation approaches:
- Combination therapy : Pairing etoposide with PARP inhibitors to exploit synthetic lethality in repair-deficient cells.
- Nanodelivery systems : Liposomal formulations to bypass ABC transporters and improve tumor targeting .
- CRISPR screens : To identify co-targetable vulnerabilities (e.g., histone deacetylases) .
Q. How do dual-targeting inhibitors (e.g., Topo II/HDAC inhibitors) enhance anticancer efficacy, and what are their design challenges?
Dual inhibitors (e.g., acridine hydroxamic acid derivatives) concurrently disrupt chromatin remodeling (via HDAC inhibition) and DNA repair (via Topo II inhibition). Design challenges include:
- Balancing selectivity : Avoiding off-target effects through structure-activity relationship (SAR) optimization.
- Pharmacokinetic optimization : Ensuring adequate blood-brain barrier penetration for CNS malignancies. Validation requires dual enzymatic inhibition assays and in vivo pharmacokinetic/pharmacodynamic (PK/PD) modeling .
Q. Methodological Guidance
Q. What in silico approaches are most effective for predicting Type II topoisomerase inhibitor binding modes?
- Molecular docking : Tools like AutoDock Vina to simulate inhibitor binding to the Topo II ATPase domain or DNA-binding cleft.
- Molecular dynamics (MD) simulations : To assess complex stability and identify critical residues (e.g., Tyr805 in human Topo IIα).
- QSAR modeling : Using datasets from NCI-60 screens to correlate chemical descriptors (e.g., logP, polar surface area) with activity .
Q. How can researchers validate the sequence specificity of Type II topoisomerase inhibitor-induced DNA cleavage?
- Ligation-mediated PCR (LM-PCR) : Maps cleavage sites at single-nucleotide resolution.
- Comparative genomic hybridization (CGH) : Identifies genomic regions prone to inhibitor-induced breaks (e.g., MLL gene loci in secondary leukemias) .
Q. Contradictions and Emerging Insights
- Paradoxical Topo IIβ roles : While Topo IIα is linked to replication, Topo IIβ is implicated in transcription and neuronal development, complicating inhibitor specificity .
- Natural product inhibitors : Flavonoids (e.g., daidzein) show Topo II inhibition but lack clinical potency due to poor bioavailability, necessitating structural derivatization .
Properties
Molecular Formula |
C18H15N3O4 |
---|---|
Molecular Weight |
337.3 g/mol |
IUPAC Name |
4-[[8-(methylamino)-2-oxo-1H-quinoline-3-carbonyl]amino]benzoic acid |
InChI |
InChI=1S/C18H15N3O4/c1-19-14-4-2-3-11-9-13(17(23)21-15(11)14)16(22)20-12-7-5-10(6-8-12)18(24)25/h2-9,19H,1H3,(H,20,22)(H,21,23)(H,24,25) |
InChI Key |
VUXYPRAXFABKBH-UHFFFAOYSA-N |
Canonical SMILES |
CNC1=CC=CC2=C1NC(=O)C(=C2)C(=O)NC3=CC=C(C=C3)C(=O)O |
Origin of Product |
United States |
Disclaimer and Information on In-Vitro Research Products
Please be aware that all articles and product information presented on BenchChem are intended solely for informational purposes. The products available for purchase on BenchChem are specifically designed for in-vitro studies, which are conducted outside of living organisms. In-vitro studies, derived from the Latin term "in glass," involve experiments performed in controlled laboratory settings using cells or tissues. It is important to note that these products are not categorized as medicines or drugs, and they have not received approval from the FDA for the prevention, treatment, or cure of any medical condition, ailment, or disease. We must emphasize that any form of bodily introduction of these products into humans or animals is strictly prohibited by law. It is essential to adhere to these guidelines to ensure compliance with legal and ethical standards in research and experimentation.