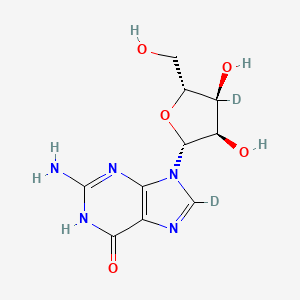
Guanosine-8-d-2
- Click on QUICK INQUIRY to receive a quote from our team of experts.
- With the quality product at a COMPETITIVE price, you can focus more on your research.
Overview
Description
Guanosine-8-d-2 (hypothetical IUPAC name: 8-deutero-2'-deoxyguanosine) is a deuterated analog of 2'-deoxyguanosine, where a hydrogen atom at the C-8 position of the guanine base is replaced with deuterium. This modification introduces isotopic labeling, which is critical for tracing metabolic pathways, studying enzymatic repair mechanisms, and analyzing DNA dynamics via techniques like nuclear magnetic resonance (NMR) or mass spectrometry.
Preparation Methods
Synthetic Routes and Reaction Conditions: The preparation of guanosine-8-d-2 can be achieved through various synthetic routes. One common method involves the use of aqueous strategies that avoid the use of protecting groups and chromatography. This method allows for the preparation of 5′-substituted guanosine derivatives . Another approach involves combinatorial metabolic engineering in Escherichia coli, where the purine synthesis pathway is overexpressed, and genes involved in guanosine catabolism are deleted to increase guanosine accumulation .
Industrial Production Methods: Industrial production of this compound primarily relies on microbial fermentation. This method is preferred due to its cost-effectiveness and ability to produce high yields. The guanosine-producing strains are optimized through metabolic engineering to enhance guanosine synthesis and accumulation .
Chemical Reactions Analysis
Types of Reactions: Guanosine-8-d-2 undergoes various chemical reactions, including oxidation, reduction, and substitution. For instance, it can be oxidized to form 8-oxo-7,8-dihydro-2′-deoxyguanosine, a common oxidative DNA damage . Additionally, guanosine can be substituted at the C8 position by free radical species generated photochemically from simple alcohols, amines, and ethers .
Common Reagents and Conditions: Common reagents used in these reactions include chloroacetyl chloride, 2-bromopropionyl bromide, and epichlorohydrin. These reagents are used to graft guanosine onto various polymers, enhancing its biological activities .
Major Products: The major products formed from these reactions include various guanosine derivatives, such as 8-oxo-7,8-dihydro-2′-deoxyguanosine and guanosine-polymer conjugates. These products have significant biological and industrial applications .
Scientific Research Applications
Cancer Research
Guanosine-8-d-2 has shown promise in cancer research, particularly in the context of oxidative DNA damage and its implications for tumorigenesis.
Oxidative Damage Biomarker
8-Hydroxy-2-deoxyguanosine (8-OHdG), a product of oxidative stress involving guanosine, is widely recognized as a biomarker for oxidative DNA damage. Elevated levels of 8-OHdG have been associated with various cancers, indicating its potential as a prognostic marker. Studies have demonstrated that higher expression levels of 8-OHdG in tumor tissues correlate with poor prognosis in solid tumors .
Study | Findings |
---|---|
Meta-analysis on 8-OHdG levels | High levels in cancer patients indicate poor prognosis . |
Urinary 8-OHdG as a biomarker | Suggests risk assessment for various cancers . |
Molecular Biology
In molecular biology, this compound is utilized to probe the conformational preferences of nucleic acids.
Stabilization of Nucleic Acids
Research indicates that modifications at the C-8 position of guanosine can enhance the stability of nucleic acid structures. For instance, 8-substituted purine nucleosides have been shown to stabilize Z-DNA forms and influence hybridization properties . This stabilization is crucial for developing novel therapeutic agents targeting specific RNA structures.
Modification | Effect on Stability |
---|---|
8-Methoxyguanosine | Decreases thermodynamic stability but increases N-glycoside bond stability . |
C8-Alkylated guanosines | Exhibits antiviral and anticancer activities . |
Medicinal Chemistry
The medicinal chemistry applications of this compound are primarily focused on its potential as an antiviral and anticancer agent.
Antiviral Properties
C8-substituted guanosine analogs have demonstrated significant antiviral activity against several viruses. For instance, 8-methyladenosine has been identified as a potent inhibitor of the vaccinia virus, while other C8-modified nucleotides show promise against respiratory syncytial virus (RSV) .
Anticancer Activity
The incorporation of this compound into therapeutic strategies is being explored for its ability to induce apoptosis in cancer cell lines. Studies have reported that these modified nucleosides can effectively inhibit the proliferation of various cancer types, including leukemias and solid tumors .
Application | Target Virus/Cancer | Mechanism |
---|---|---|
8-Methyladenosine | Vaccinia virus | Inhibition of viral replication . |
C8-Alkylated GTP analogs | Bacterial infections | Inhibition of FtsZ polymerization . |
Case Studies
Several case studies highlight the effectiveness of this compound in clinical and laboratory settings:
- Prognostic Marker in Cancer : A study involving over 800 patients showed that high urinary levels of 8-OHdG were significantly associated with increased cancer risk and poor patient outcomes, reinforcing its role as a reliable biomarker for oxidative stress-related cancers .
- Therapeutic Development : Research on C8-modified guanosine derivatives has led to the development of new antibiotics targeting bacterial infections, showcasing their potential beyond traditional antiviral applications .
Conclusions
Mechanism of Action
The mechanism of action of guanosine-8-d-2 involves its interaction with various molecular targets and pathways. In the central nervous system, guanosine acts as a neuromodulator, mediating cellular processes such as cell growth, differentiation, and survival. It exerts protective effects in models of neurotoxicity and neurological disorders by modulating glutamate uptake, decreasing the production of reactive oxygen species, improving mitochondrial function, and presenting anti-inflammatory properties . Guanosine also promotes neural stem cell proliferation and neuronal differentiation .
Comparison with Similar Compounds
Comparison with Structurally Related Compounds
Structural and Chemical Properties
The C-8 position of guanine is a hotspot for chemical modifications due to its susceptibility to oxidation, alkylation, and halogenation. Below is a comparative analysis of Guanosine-8-d-2 and key analogs:
Compound | Substituent at C-8 | Molecular Weight (g/mol) | Key Properties |
---|---|---|---|
This compound | Deuterium (²H) | 268.25* | Isotopic labeling; minimal steric/electronic perturbation. |
8-OHdG | Hydroxyl (-OH) | 283.24 | Oxidative damage biomarker; induces DNA helix distortion and mispairing. |
8-Br-dG | Bromine (Br) | 326.12 | Bulky substituent; inhibits DNA polymerases; used in photodynamic therapy. |
N-(deoxyguanosin-8-yl)-N-acetyl-2-aminofluorene | Aromatic adduct | ~455.40 | Carcinogen-DNA adduct; causes localized DNA denaturation. |
*Calculated based on 2'-deoxyguanosine (267.24 g/mol) + deuterium substitution.
Key Observations :
- This compound: The deuterium substitution minimally alters the molecule’s physical properties, making it ideal for isotopic tracing without disrupting native DNA interactions .
- 8-OHdG : The hydroxyl group introduces significant polarity, leading to DNA helix destabilization and G→T transversion mutations during replication .
- 8-Br-dG : Bromine’s electronegativity and size sterically hinder DNA polymerase activity, enabling its use as a chain terminator .
- C-8 adducts (e.g., N-acetyl-2-aminofluorene): These bulky adducts induce single-stranded DNA regions, making them substrates for repair enzymes like S1 nuclease .
This compound
- Isotopic Labeling : Enables precise tracking of nucleotide metabolism and repair kinetics in deuterium NMR studies.
- Enzyme Interaction Studies : Used to investigate kinetic isotope effects in enzymes like DNA glycosylases .
8-OHdG
- Biomarker for Oxidative Stress : Elevated levels correlate with aging, cancer, and neurodegenerative diseases. Detection methods include ELISA and HPLC .
- Mutagenicity : Induces replication errors, serving as a model for studying oxidative DNA damage .
8-Br-dG
- Therapeutic Applications : Used in photodynamic therapy due to bromine’s photosensitizing properties.
- DNA Synthesis Inhibition : Acts as a chain terminator in sequencing and polymerase studies .
Impact on DNA Structure and Repair
- This compound: Deuterium’s small size preserves B-DNA conformation, unlike bulkier adducts. Enzymatic repair mechanisms (e.g., base excision repair) are likely unaffected .
- 8-OHdG : Causes significant helical distortion, triggering repair by OGG1 glycosylase. Unrepaired lesions lead to mutations .
- C-8 Adducts (e.g., N-acetyl-2-aminofluorene): Major helix distortions make these adducts targets for single-strand-specific nucleases like S1 and Neurospora crassa endonuclease .
Biological Activity
Guanosine-8-d-2, also known as 8-oxoguanosine, is an oxidized derivative of guanosine that plays a significant role in various biological processes, particularly in the context of oxidative stress and DNA damage. This article explores the biological activity of this compound, focusing on its mechanisms of action, implications in diseases, and relevant research findings.
Chemical Structure and Properties
This compound is characterized by the presence of an oxygen atom at the C8 position of the guanine base, making it susceptible to further reactions that can lead to mutagenic outcomes. This compound is a product of oxidative DNA damage and serves as a marker for oxidative stress in biological systems.
- Oxidative Stress Response : this compound is formed primarily through the reaction of guanine with reactive oxygen species (ROS). It is one of the most prevalent oxidative lesions in DNA, which can lead to mutations if not repaired properly. The presence of 8-oxoguanosine can disrupt normal base pairing during DNA replication, leading to G>T transversions, a common mutation found in various cancers .
- DNA Repair Pathways : The body employs several DNA repair mechanisms to counteract the effects of oxidative damage. The enzyme 8-oxoguanine DNA glycosylase (OGG1) specifically recognizes and excises 8-oxoguanine from DNA, preventing potential mutations . This repair process is crucial for maintaining genomic stability.
1. Cancer
Research has demonstrated a strong correlation between elevated levels of this compound and various types of cancer. For instance, studies indicate that increased oxidative DNA damage markers, including 8-OHdG (a derivative), are associated with tumor progression and poor prognosis in cancers such as lung, breast, and colorectal cancer .
2. Cardiovascular Disease
Elevated levels of 8-OHdG have also been linked to cardiovascular diseases (CVD). A meta-analysis revealed that CVD patients exhibit significantly higher levels of 8-OHdG compared to healthy controls, suggesting its potential role as a biomarker for assessing cardiovascular risk .
3. Neurological Disorders
The accumulation of oxidative DNA damage is implicated in neurodegenerative diseases such as Alzheimer's and Parkinson's disease. Increased levels of this compound have been observed in brain tissues affected by these conditions, indicating its involvement in neurodegeneration .
Table 1: Summary of Research Findings on this compound
Future Directions for Research
The ongoing research into this compound highlights its potential as a biomarker for various diseases related to oxidative stress. Future studies should focus on:
- Developing more sensitive assays for detecting 8-OHdG levels in clinical settings.
- Investigating the therapeutic implications of targeting oxidative stress pathways.
- Exploring the role of this compound in epigenetic regulation and gene expression.
Q & A
Basic Research Questions
Q. What are the primary methodologies for synthesizing and characterizing Guanosine-8-d-2 in laboratory settings?
Synthesis typically involves isotopic labeling via enzymatic or chemical methods, with deuterium incorporation at the 8th position of guanosine. Characterization requires nuclear magnetic resonance (NMR) to confirm deuterium placement and high-performance liquid chromatography (HPLC) to assess purity. For reproducibility, experimental protocols must detail reaction conditions (e.g., temperature, pH, catalysts) and purification steps . Researchers should cross-validate results using mass spectrometry (MS) and compare retention times with non-deuterated guanosine standards .
Q. How can researchers validate the isotopic purity of this compound in experimental systems?
Isotopic purity is critical for minimizing confounding variables. Methodologies include:
- NMR spectroscopy : Analyze peak splitting patterns to confirm deuterium substitution at the 8th position.
- Isotope-ratio mass spectrometry (IRMS) : Quantify deuterium-to-hydrogen ratios with precision ≥99% .
- Stability testing : Monitor isotopic integrity under experimental conditions (e.g., temperature, solvent systems) to rule out deuterium exchange .
Q. What are the standard protocols for incorporating this compound into nucleotide stability studies?
Design experiments to assess:
- Thermodynamic stability : Use differential scanning calorimetry (DSC) to compare melting points with non-deuterated analogs.
- Hydrolytic resistance : Perform kinetic assays under acidic/alkaline conditions, measuring degradation via UV-Vis spectroscopy .
Document all parameters (e.g., buffer composition, incubation times) to enable replication .
Advanced Research Questions
Q. How do isotopic effects of deuterium at the 8th position influence guanosine’s interaction with RNA polymerases or DNA helicases?
Advanced studies require:
- Kinetic isotope effect (KIE) analysis : Compare reaction rates (e.g., kcat) between deuterated and non-deuterated substrates in enzymatic assays .
- Molecular dynamics (MD) simulations : Model how deuterium alters hydrogen bonding or steric hindrance in enzyme active sites .
Contradictions in KIE data (e.g., unexpected rate enhancements) should prompt re-evaluation of solvent deuteration levels or enzyme preparation methods .
Q. What experimental designs address contradictions in reported deuterium isotope effects on guanosine’s base-pairing fidelity?
Conflicting data often arise from variations in experimental conditions. Mitigate this by:
- Controlled replication : Use standardized buffers (e.g., 10 mM Tris-HCl, pH 7.4) and rigorously deionized solvents.
- Multivariate analysis : Apply ANOVA to isolate variables (e.g., temperature, ionic strength) contributing to discrepancies .
- Cross-disciplinary validation : Compare results across techniques (e.g., X-ray crystallography, NMR) to resolve ambiguities .
Q. How can researchers optimize this compound for tracer studies in metabolic flux analysis?
Advanced applications require:
- Tracer design : Ensure isotopic labeling does not interfere with metabolic pathways (validate via LC-MS/MS profiling of downstream metabolites).
- Quantitative modeling : Use compartmental flux models to distinguish isotopic dilution effects from true metabolic rates .
Include negative controls (e.g., non-deuterated guanosine) to confirm tracer specificity .
Q. Methodological Frameworks
Q. What frameworks guide hypothesis formulation for this compound studies in enzymology or structural biology?
Adopt the FINER criteria (Feasible, Interesting, Novel, Ethical, Relevant) to structure research questions. For example:
- Feasibility: Assess access to deuterated precursors and specialized instrumentation (e.g., cryo-EM for structural studies).
- Novelty: Identify gaps in existing literature, such as unresolved KIE mechanisms in ribozyme catalysis .
Q. How should researchers document and report contradictory data in studies involving this compound?
Follow guidelines for transparent reporting:
- Data availability statements : Share raw spectra, chromatograms, and simulation files as supplementary materials .
- Error analysis : Quantify uncertainties in isotopic purity measurements (e.g., ±0.5% via IRMS) and disclose instrument calibration protocols .
- Peer review alignment : Preemptively address potential critiques (e.g., solvent effects on deuterium retention) in the discussion section .
Q. Tables for Reference
Parameter | Method | Acceptable Range | Citation |
---|---|---|---|
Isotopic Purity | IRMS | ≥99% D/H ratio | |
NMR Confirmation | 1H-NMR (400 MHz) | Single peak at δ 8.2 ppm | |
Degradation Kinetics (pH 7) | UV-Vis (260 nm) | t1/2 ≥ 48 hrs |
Properties
Molecular Formula |
C10H13N5O5 |
---|---|
Molecular Weight |
285.25 g/mol |
IUPAC Name |
2-amino-8-deuterio-9-[(2R,3R,4S,5R)-4-deuterio-3,4-dihydroxy-5-(hydroxymethyl)oxolan-2-yl]-1H-purin-6-one |
InChI |
InChI=1S/C10H13N5O5/c11-10-13-7-4(8(19)14-10)12-2-15(7)9-6(18)5(17)3(1-16)20-9/h2-3,5-6,9,16-18H,1H2,(H3,11,13,14,19)/t3-,5-,6-,9-/m1/s1/i2D,5D |
InChI Key |
NYHBQMYGNKIUIF-VXBMHFSWSA-N |
Isomeric SMILES |
[2H]C1=NC2=C(N1[C@H]3[C@@H]([C@]([C@H](O3)CO)([2H])O)O)N=C(NC2=O)N |
Canonical SMILES |
C1=NC2=C(N1C3C(C(C(O3)CO)O)O)N=C(NC2=O)N |
Origin of Product |
United States |
Disclaimer and Information on In-Vitro Research Products
Please be aware that all articles and product information presented on BenchChem are intended solely for informational purposes. The products available for purchase on BenchChem are specifically designed for in-vitro studies, which are conducted outside of living organisms. In-vitro studies, derived from the Latin term "in glass," involve experiments performed in controlled laboratory settings using cells or tissues. It is important to note that these products are not categorized as medicines or drugs, and they have not received approval from the FDA for the prevention, treatment, or cure of any medical condition, ailment, or disease. We must emphasize that any form of bodily introduction of these products into humans or animals is strictly prohibited by law. It is essential to adhere to these guidelines to ensure compliance with legal and ethical standards in research and experimentation.