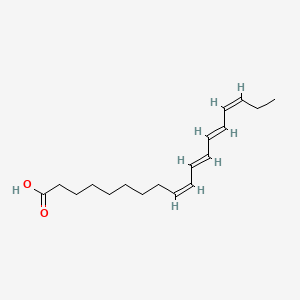
cis-Parinaric acid
Overview
Description
Cis-Parinaric acid: is a naturally occurring polyunsaturated fatty acid with an unusual conjugated (Z,E,E,Z) tetraene structure. It was initially developed as a membrane probe by Hudson and co-workers in 1975 . Among currently available fluorescent probes, this compound is the closest structural analog to intrinsic membrane lipids. Its chemical and physical properties have been well characterized.
Preparation Methods
Synthetic Routes:: The synthesis of cis-Parinaric acid involves specific chemical reactions to introduce the conjugated tetraene structure. While I don’t have specific synthetic routes at hand, researchers typically achieve its synthesis through multi-step processes.
Industrial Production:: Industrial-scale production methods for this compound are not widely documented. its natural occurrence in the seeds of the Makita tree (a tropical rainforest tree indigenous to Fiji) suggests that extraction from natural sources might be a viable approach .
Chemical Reactions Analysis
Photochemical Dimerization
cis-Parinaric acid undergoes second-order photochemical dimerization in lipid bilayers when exposed to intense illumination. This reaction is characterized by:
-
Loss of the original absorption maxima (~304 nm and ~320 nm) and fluorescence (λ<sub>ex</sub>/λ<sub>em</sub> = 304/416 nm) due to conjugation disruption .
-
Formation of a triene chromophore with new absorption bands, detectable via HPLC and fluorescence quenching .
Key Factors Influencing Dimerization:
Mechanism : The reaction proceeds via a diffusion-controlled process, where the lipid bilayer’s physical state governs the collision frequency of this compound molecules .
Oxidation Reactions
The conjugated tetraene system makes this compound highly susceptible to peroxyl radical-induced oxidation , enabling its use as a peroxidation sensor:
-
Pro-oxidant systems (e.g., AAPH/AMVN) generate peroxyl radicals that oxidize the tetraene chain, reducing fluorescence intensity by ~55% within 60 minutes .
-
Antioxidants like amphotericin B inhibit oxidation by scavenging radicals, reducing fluorescence loss by 20–40% at equimolar ratios .
Oxidation Parameters:
Initiator | System | Half-life (min) | Inhibition by Amphotericin B | Citation |
---|---|---|---|---|
AAPH | PnA-human serum albumin | 38 ± 1 | 54 ± 4 (20 µM AmB) | |
AMVN | Liposomal PnA | 25 ± 2 | 45 ± 3 (10 µM AmB) |
Storage Stability : Solutions degrade within hours under aerobic conditions but remain stable for >6 months when stored at -20°C under argon .
Incorporation into Membrane Lipids
In biological systems, this compound integrates into phospholipids via enzymatic esterification:
-
Primary incorporation : 71% into phosphatidylcholine and 20% into phosphatidylethanolamine in synaptosomal membranes .
-
Requirements : Mg<sup>2+</sup>/Ca<sup>2+</sup>, ATP, and coenzyme A enhance incorporation efficiency .
Phospholipid Distribution:
Phospholipid | Incorporation (%) | Membrane Type | Citation |
---|---|---|---|
Phosphatidylcholine | 71 | Synaptosomal | |
Phosphatidylethanolamine | 20 | Microsomal | |
Phosphatidylserine | <2 | Synaptosomal |
Interaction with Lipid Bilayers
Molecular dynamics simulations reveal:
-
Orientation : Carboxylate group faces the lipid-water interface, while the tetraene tail spans the bilayer leaflet .
-
Partitioning : Preferentially localizes in disordered lipid phases (e.g., POPC) but shows reduced partitioning in gel-phase DPPC compared to trans-parinaric acid .
Depth-Dependent Localization:
Lipid Phase | Probe Depth (Å from bilayer center) | Citation |
---|---|---|
POPC | 12–14 (cis-PnA) vs. 16–18 (trans-PnA) | |
DPPC | 14–16 (both isomers) |
Fluorescence Quenching by Amphiphiles
This compound’s fluorescence is quenched collisionaly by membrane-bound amphiphiles (e.g., amphotericin B):
Scientific Research Applications
Marker for Lipid Peroxidation
Cis-parinaric acid is widely recognized as a sensitive fluorescent probe for detecting lipid peroxidation in biological systems. Its incorporation into biomembranes allows researchers to monitor oxidative stress and the early stages of lipid peroxidation. Studies have demonstrated that this compound can be either inserted freely into membranes or incorporated into lipids, making it a valuable tool for assessing oxidative damage in eukaryotic cells .
Key Findings:
- Higher Peroxidation Rates: Research indicates that the peroxidation rate of this compound surpasses that of other polyunsaturated fatty acids, such as arachidonic and linoleic acids .
- Fluorescence Monitoring: The fluorescence intensity of this compound decreases upon reaction with free radicals, allowing for real-time monitoring of oxidative processes .
Cytotoxicity in Cancer Research
This compound exhibits selective cytotoxic effects on malignant cells while sparing normal cells. Studies have shown that it can significantly induce cell death in various glioma cell lines without adversely affecting normal astrocytes. This property positions this compound as a potential candidate for developing novel chemotherapeutic agents targeting gliomas .
Case Study:
- Malignant Glioma Cells: In vitro studies revealed that concentrations of this compound that caused nearly 90% toxicity in glioma cultures resulted in minimal toxicity to normal astrocytes. The mechanism behind this selective cytotoxicity appears to be related to oxidative stress induced by lipid peroxidation .
Incorporation into Membrane Phospholipids
This compound is incorporated into membrane phospholipids, particularly in brain tissues, where it serves as a fluorescent marker for studying membrane dynamics and lipid interactions. This property facilitates investigations into membrane-mediated processes and the behavior of fatty acids within lipid bilayers .
Research Highlights:
- Fluorescent Probes: The incorporation of this compound into phosphatidylcholine and phosphatidylethanolamine has been documented, demonstrating its utility as a probe for studying membrane characteristics .
- Membrane Dynamics: Studies utilizing molecular dynamics simulations have shown how this compound affects lipid bilayer properties, providing insights into its role in membrane fluidity and organization .
Applications in Antioxidant Studies
This compound has been employed extensively in evaluating the efficacy of antioxidants. Its ability to undergo oxidation while emitting fluorescence makes it an ideal candidate for assays designed to measure antioxidant activity and lipid peroxidation levels in biological samples .
Experimental Uses:
- Antioxidant Evaluation: Researchers utilize this compound to assess the protective effects of various compounds against oxidative damage by measuring changes in fluorescence intensity .
- Peroxidation Assays: It is also used to study the relationship between lipid peroxidation and apoptosis, contributing to our understanding of cell death mechanisms .
Future Directions and Research Opportunities
The unique properties of this compound open avenues for further research across multiple disciplines:
- Cancer Therapeutics: Continued exploration into its selective cytotoxicity could lead to innovative treatments for various cancers.
- Neuroscience Applications: Its role as a fluorescent probe may enhance our understanding of neurological processes and diseases linked to oxidative stress.
- Lipid Metabolism Studies: Investigating its incorporation into different lipid classes could provide insights into metabolic pathways involving polyunsaturated fatty acids.
Mechanism of Action
The exact mechanism by which cis-Parinaric acid exerts its effects remains an active area of study. It likely involves interactions with cellular membranes, lipid peroxidation pathways, and oxidative stress responses.
Comparison with Similar Compounds
Cis-Parinaric acid stands out due to its conjugated tetraene structure. Similar compounds include other polyunsaturated fatty acids, but none precisely match its unique properties.
Biological Activity
Cis-parinaric acid (c-PnA) is a conjugated fatty acid known for its unique structural properties and biological activities. It has gained attention in scientific research for its potential applications in biochemistry, cell biology, and medicine. This article explores the biological activity of c-PnA, focusing on its effects on cellular processes, cytotoxicity, and its role as a fluorescent probe.
Structure and Properties
This compound is characterized by four conjugated double bonds in its long hydrocarbon chain. Its molecular structure allows it to integrate into lipid membranes, influencing membrane dynamics and properties. The compound exists in two isomeric forms: this compound (c-PnA) and trans-parinaric acid (t-PnA), with the former exhibiting distinct biological behaviors due to its non-linear configuration.
1. Cytotoxicity
Research indicates that c-PnA exhibits significant cytotoxic effects on malignant cells, particularly glioma cells. Notably, studies have shown that c-PnA can induce cell death at concentrations that do not harm normal astrocytes, suggesting a selective toxicity towards cancerous cells.
- Case Study : A study published in Cancer Research demonstrated that c-PnA effectively reduced the viability of cultured malignant glioma cells while sparing normal astrocytes. The mechanism appears to involve direct interaction with cellular membranes, leading to altered membrane integrity and subsequent apoptosis in cancer cells .
Study | Cell Type | Concentration | Effect |
---|---|---|---|
Malignant Gliomas | Varies | Significant cytotoxicity | |
Various Cancer Lines | Low Concentrations | Selective apoptosis |
2. Membrane Dynamics
This compound serves as a useful fluorescent probe for studying lipid bilayer dynamics. Molecular dynamics simulations have shown that c-PnA can influence the order and fluidity of lipid membranes.
- Research Findings : In a comparative study using molecular dynamics simulations, it was found that c-PnA induces disorder in lipid bilayers compared to its trans counterpart. This behavior is attributed to the unique configuration of c-PnA, which affects how it interacts with lipid chains .
Parameter | c-PnA | t-PnA |
---|---|---|
Membrane Order | Decreases | Increases |
Diffusion Rate | Slower due to chain kinks | Faster due to linear structure |
3. Fluorescent Properties
This compound is utilized as a fluorescent probe due to its ability to emit fluorescence upon incorporation into lipid membranes. This property makes it valuable for studying membrane dynamics and the effects of various treatments on cellular membranes.
Q & A
Basic Research Questions
Q. What are the key optical properties of cis-Parinaric acid that make it suitable as a fluorescent probe in lipid peroxidation studies?
this compound exhibits a large Stokes shift (~100 nm), enabling minimal interference from excitation light during fluorescence detection. Its absorption spectrum includes peaks at ~300 nm and 320 nm with high extinction coefficients, making it sensitive to environmental changes. Fluorescence is quenched upon oxidation, allowing direct correlation between signal loss and lipid peroxidation severity. Its near-zero fluorescence in aqueous environments ensures specificity for lipid-rich systems .
Q. How should this compound be stored and handled to prevent degradation during experiments?
Store at ≤–20°C in dark, airtight containers with 10 µg/mL BHT to inhibit oxidation. Prepare working solutions under inert gas (e.g., nitrogen) using degassed solvents. Avoid prolonged light exposure to prevent photodimerization. Discard unused solutions immediately after experiments, as room-temperature instability accelerates degradation .
Q. What methodologies are recommended for preparing this compound working solutions to ensure consistent experimental results?
Dissolve in ethanol or methanol under nitrogen atmosphere. Use degassed buffers (e.g., PBS) for dilution. Centrifuge before use to remove insoluble oxidized byproducts. Validate solubility via fluorescence baseline measurements in target solvents .
Advanced Research Questions
Q. What experimental design considerations are critical when using this compound to quantify lipid peroxidation in live cell assays?
- Cell viability: Optimize incubation time (e.g., ≤5 hours) to avoid cytotoxicity from prolonged oxidative stress .
- Oxidation controls: Include BHT-supplemented samples to distinguish baseline fluorescence loss from experimental peroxidation.
- Real-time monitoring: Use flow cytometry (FACS) for dynamic quantification, as static endpoint measurements may miss transient peroxidation events .
Q. How can researchers resolve discrepancies in fluorescence intensity data obtained from this compound in different lipid environments?
- Calibration curves: Generate lipid-specific standards (e.g., liposomes with defined fatty acid compositions) to account for microenvironmental quenching effects.
- Interference checks: Measure background fluorescence in lipid-free buffers and subtract from experimental values.
- Parallel assays: Validate results with orthogonal methods (e.g., thiobarbituric acid reactive substances assay) .
Q. What are the limitations of using this compound in long-term oxidative stress studies, and how can they be mitigated?
- Photostability: Prolonged light exposure causes photodimerization; use low-intensity excitation and shielded setups.
- Auto-oxidation: Even inert storage leads to gradual degradation; validate batch stability via absorption spectra before use.
- Cellular uptake variability: Normalize fluorescence data to cell membrane lipid content (e.g., via co-staining with non-oxidizable dyes) .
Q. How does the conjugation state of this compound influence its interactions with membrane lipids, and how can this be experimentally validated?
The (Z,E,E,Z)-tetraene structure allows this compound to integrate into lipid bilayers similarly to natural unsaturated fatty acids. To validate:
- Fluorescence anisotropy: Compare membrane fluidity changes in synthetic vesicles with/without this compound.
- Quenching assays: Use brominated lipids to assess proximity to specific membrane regions .
Q. What controls should be included when using this compound to distinguish between specific lipid peroxidation and non-specific oxidation artifacts?
Properties
IUPAC Name |
(9Z,11E,13E,15Z)-octadeca-9,11,13,15-tetraenoic acid | |
---|---|---|
Source | PubChem | |
URL | https://pubchem.ncbi.nlm.nih.gov | |
Description | Data deposited in or computed by PubChem | |
InChI |
InChI=1S/C18H28O2/c1-2-3-4-5-6-7-8-9-10-11-12-13-14-15-16-17-18(19)20/h3-10H,2,11-17H2,1H3,(H,19,20)/b4-3-,6-5+,8-7+,10-9- | |
Source | PubChem | |
URL | https://pubchem.ncbi.nlm.nih.gov | |
Description | Data deposited in or computed by PubChem | |
InChI Key |
IJTNSXPMYKJZPR-ZSCYQOFPSA-N | |
Source | PubChem | |
URL | https://pubchem.ncbi.nlm.nih.gov | |
Description | Data deposited in or computed by PubChem | |
Canonical SMILES |
CCC=CC=CC=CC=CCCCCCCCC(=O)O | |
Source | PubChem | |
URL | https://pubchem.ncbi.nlm.nih.gov | |
Description | Data deposited in or computed by PubChem | |
Isomeric SMILES |
CC/C=C\C=C\C=C\C=C/CCCCCCCC(=O)O | |
Source | PubChem | |
URL | https://pubchem.ncbi.nlm.nih.gov | |
Description | Data deposited in or computed by PubChem | |
Molecular Formula |
C18H28O2 | |
Source | PubChem | |
URL | https://pubchem.ncbi.nlm.nih.gov | |
Description | Data deposited in or computed by PubChem | |
DSSTOX Substance ID |
DTXSID501027236 | |
Record name | (9Z,11E,13E,15Z)-9,11,13,15-Octadecatetraenoic acid | |
Source | EPA DSSTox | |
URL | https://comptox.epa.gov/dashboard/DTXSID501027236 | |
Description | DSSTox provides a high quality public chemistry resource for supporting improved predictive toxicology. | |
Molecular Weight |
276.4 g/mol | |
Source | PubChem | |
URL | https://pubchem.ncbi.nlm.nih.gov | |
Description | Data deposited in or computed by PubChem | |
CAS No. |
593-38-4 | |
Record name | cis-Parinaric acid | |
Source | CAS Common Chemistry | |
URL | https://commonchemistry.cas.org/detail?cas_rn=593-38-4 | |
Description | CAS Common Chemistry is an open community resource for accessing chemical information. Nearly 500,000 chemical substances from CAS REGISTRY cover areas of community interest, including common and frequently regulated chemicals, and those relevant to high school and undergraduate chemistry classes. This chemical information, curated by our expert scientists, is provided in alignment with our mission as a division of the American Chemical Society. | |
Explanation | The data from CAS Common Chemistry is provided under a CC-BY-NC 4.0 license, unless otherwise stated. | |
Record name | cis-Parinaric acid | |
Source | ChemIDplus | |
URL | https://pubchem.ncbi.nlm.nih.gov/substance/?source=chemidplus&sourceid=0000593384 | |
Description | ChemIDplus is a free, web search system that provides access to the structure and nomenclature authority files used for the identification of chemical substances cited in National Library of Medicine (NLM) databases, including the TOXNET system. | |
Record name | (9Z,11E,13E,15Z)-9,11,13,15-Octadecatetraenoic acid | |
Source | EPA DSSTox | |
URL | https://comptox.epa.gov/dashboard/DTXSID501027236 | |
Description | DSSTox provides a high quality public chemistry resource for supporting improved predictive toxicology. | |
Record name | PARINARIC ACID, (Z)- | |
Source | FDA Global Substance Registration System (GSRS) | |
URL | https://gsrs.ncats.nih.gov/ginas/app/beta/substances/KM4KXM284R | |
Description | The FDA Global Substance Registration System (GSRS) enables the efficient and accurate exchange of information on what substances are in regulated products. Instead of relying on names, which vary across regulatory domains, countries, and regions, the GSRS knowledge base makes it possible for substances to be defined by standardized, scientific descriptions. | |
Explanation | Unless otherwise noted, the contents of the FDA website (www.fda.gov), both text and graphics, are not copyrighted. They are in the public domain and may be republished, reprinted and otherwise used freely by anyone without the need to obtain permission from FDA. Credit to the U.S. Food and Drug Administration as the source is appreciated but not required. | |
Retrosynthesis Analysis
AI-Powered Synthesis Planning: Our tool employs the Template_relevance Pistachio, Template_relevance Bkms_metabolic, Template_relevance Pistachio_ringbreaker, Template_relevance Reaxys, Template_relevance Reaxys_biocatalysis model, leveraging a vast database of chemical reactions to predict feasible synthetic routes.
One-Step Synthesis Focus: Specifically designed for one-step synthesis, it provides concise and direct routes for your target compounds, streamlining the synthesis process.
Accurate Predictions: Utilizing the extensive PISTACHIO, BKMS_METABOLIC, PISTACHIO_RINGBREAKER, REAXYS, REAXYS_BIOCATALYSIS database, our tool offers high-accuracy predictions, reflecting the latest in chemical research and data.
Strategy Settings
Precursor scoring | Relevance Heuristic |
---|---|
Min. plausibility | 0.01 |
Model | Template_relevance |
Template Set | Pistachio/Bkms_metabolic/Pistachio_ringbreaker/Reaxys/Reaxys_biocatalysis |
Top-N result to add to graph | 6 |
Feasible Synthetic Routes
Disclaimer and Information on In-Vitro Research Products
Please be aware that all articles and product information presented on BenchChem are intended solely for informational purposes. The products available for purchase on BenchChem are specifically designed for in-vitro studies, which are conducted outside of living organisms. In-vitro studies, derived from the Latin term "in glass," involve experiments performed in controlled laboratory settings using cells or tissues. It is important to note that these products are not categorized as medicines or drugs, and they have not received approval from the FDA for the prevention, treatment, or cure of any medical condition, ailment, or disease. We must emphasize that any form of bodily introduction of these products into humans or animals is strictly prohibited by law. It is essential to adhere to these guidelines to ensure compliance with legal and ethical standards in research and experimentation.