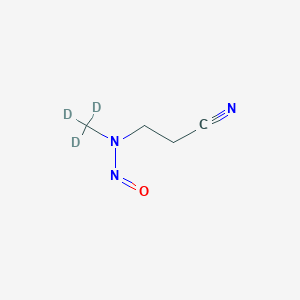
3-(Methyl-nitrosoamino)propionitrile-d3
- Click on QUICK INQUIRY to receive a quote from our team of experts.
- With the quality product at a COMPETITIVE price, you can focus more on your research.
Overview
Description
3-(Methyl-nitrosoamino)propionitrile-d3 is a deuterium-labeled compound, specifically the deuterium-labeled version of 3-(Methyl-nitrosoamino)propionitrile . Deuterium labeling involves replacing hydrogen atoms with deuterium, a stable isotope of hydrogen, which can significantly affect the pharmacokinetic and metabolic profiles of the compound . This compound is primarily used in scientific research as a tracer for quantitation during the drug development process .
Chemical Reactions Analysis
3-(Methyl-nitrosoamino)propionitrile-d3, like its non-deuterated counterpart, can undergo various chemical reactions, including:
Oxidation: This reaction involves the addition of oxygen or the removal of hydrogen. Common oxidizing agents include potassium permanganate and hydrogen peroxide.
Reduction: This reaction involves the addition of hydrogen or the removal of oxygen. Common reducing agents include lithium aluminum hydride and sodium borohydride.
Substitution: This reaction involves the replacement of one atom or group of atoms with another. Common reagents for substitution reactions include halogens and nucleophiles.
The major products formed from these reactions depend on the specific conditions and reagents used .
Scientific Research Applications
3-(Methyl-nitrosoamino)propionitrile-d3 has several applications in scientific research, including:
Chemistry: Used as a tracer in the study of reaction mechanisms and kinetics.
Biology: Employed in metabolic studies to track the incorporation and transformation of the compound in biological systems.
Medicine: Utilized in drug development to study the pharmacokinetics and metabolism of potential therapeutic agents.
Industry: Applied in the development of new materials and chemical processes.
Mechanism of Action
The mechanism of action of 3-(Methyl-nitrosoamino)propionitrile-d3 is primarily related to its role as a tracer in scientific research. The incorporation of deuterium atoms allows researchers to track the compound’s behavior in various systems, providing valuable insights into its pharmacokinetics and metabolism . The molecular targets and pathways involved depend on the specific application and the system being studied.
Comparison with Similar Compounds
3-(Methyl-nitrosoamino)propionitrile-d3 is unique due to its deuterium labeling, which distinguishes it from its non-deuterated counterpart, 3-(Methyl-nitrosoamino)propionitrile . Other similar compounds include:
3-(Methyl-nitrosoamino)propionitrile: The non-deuterated version of the compound.
3-(Methyl-d3-nitrosoamino)propionitrile: Another deuterium-labeled version with a different isotopic enrichment.
These compounds share similar chemical structures but differ in their isotopic composition, which can affect their behavior in various applications .
Properties
Molecular Formula |
C4H7N3O |
---|---|
Molecular Weight |
116.14 g/mol |
IUPAC Name |
N-(2-cyanoethyl)-N-(trideuteriomethyl)nitrous amide |
InChI |
InChI=1S/C4H7N3O/c1-7(6-8)4-2-3-5/h2,4H2,1H3/i1D3 |
InChI Key |
HZDLDFBKYBGNHG-FIBGUPNXSA-N |
Isomeric SMILES |
[2H]C([2H])([2H])N(CCC#N)N=O |
Canonical SMILES |
CN(CCC#N)N=O |
Origin of Product |
United States |
Disclaimer and Information on In-Vitro Research Products
Please be aware that all articles and product information presented on BenchChem are intended solely for informational purposes. The products available for purchase on BenchChem are specifically designed for in-vitro studies, which are conducted outside of living organisms. In-vitro studies, derived from the Latin term "in glass," involve experiments performed in controlled laboratory settings using cells or tissues. It is important to note that these products are not categorized as medicines or drugs, and they have not received approval from the FDA for the prevention, treatment, or cure of any medical condition, ailment, or disease. We must emphasize that any form of bodily introduction of these products into humans or animals is strictly prohibited by law. It is essential to adhere to these guidelines to ensure compliance with legal and ethical standards in research and experimentation.