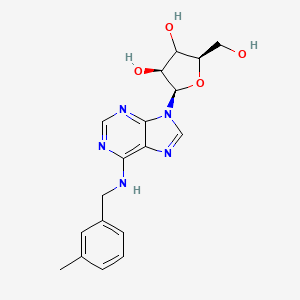
Antiviral agent 23
- Click on QUICK INQUIRY to receive a quote from our team of experts.
- With the quality product at a COMPETITIVE price, you can focus more on your research.
Overview
Description
Antiviral agent 23 is a synthetic compound designed to inhibit the replication of various viruses. It has shown broad-spectrum antiviral activity and is being studied for its potential use in treating viral infections such as influenza, hepatitis, and coronaviruses. The compound is part of a class of antiviral agents that target specific viral enzymes or proteins, disrupting the viral life cycle and preventing the spread of infection.
Preparation Methods
Synthetic Routes and Reaction Conditions
The synthesis of Antiviral agent 23 typically involves multiple steps, starting from simple organic molecules. One common synthetic route includes the following steps:
Formation of the Core Structure: The core structure of this compound is synthesized through a series of condensation reactions involving aromatic aldehydes and amines. These reactions are usually carried out in the presence of a catalyst such as hydrochloric acid or sulfuric acid.
Functional Group Modifications: The core structure is then modified by introducing various functional groups through substitution reactions. Common reagents used in these reactions include halogens, alkylating agents, and acylating agents.
Purification and Isolation: The final product is purified using techniques such as recrystallization, column chromatography, or high-performance liquid chromatography.
Industrial Production Methods
In an industrial setting, the production of this compound is scaled up using continuous flow reactors and automated synthesis platforms. These methods allow for precise control over reaction conditions, leading to higher yields and purity of the final product. The use of green chemistry principles, such as solvent recycling and energy-efficient processes, is also emphasized to minimize environmental impact.
Chemical Reactions Analysis
Types of Reactions
Antiviral agent 23 undergoes various chemical reactions, including:
Oxidation: The compound can be oxidized using reagents such as potassium permanganate or hydrogen peroxide, leading to the formation of oxidized derivatives.
Reduction: Reduction reactions can be carried out using reducing agents like sodium borohydride or lithium aluminum hydride, resulting in reduced forms of the compound.
Substitution: Substitution reactions involve the replacement of functional groups with other groups, using reagents such as halogens or alkylating agents.
Common Reagents and Conditions
Oxidation: Potassium permanganate, hydrogen peroxide; acidic or basic conditions.
Reduction: Sodium borohydride, lithium aluminum hydride; typically carried out in anhydrous solvents.
Substitution: Halogens, alkylating agents; often performed in the presence of a catalyst or under reflux conditions.
Major Products Formed
The major products formed from these reactions include various derivatives of this compound, each with unique properties and potential applications. These derivatives are often studied for their enhanced antiviral activity or reduced toxicity.
Scientific Research Applications
Antiviral agent 23 has a wide range of scientific research applications, including:
Chemistry: Used as a model compound for studying reaction mechanisms and developing new synthetic methodologies.
Biology: Investigated for its ability to inhibit viral replication in cell culture and animal models.
Medicine: Potential therapeutic agent for treating viral infections such as influenza, hepatitis, and coronaviruses.
Industry: Utilized in the development of antiviral coatings and materials for medical devices and personal protective equipment.
Mechanism of Action
The mechanism of action of Antiviral agent 23 involves the inhibition of viral enzymes or proteins essential for viral replication. The compound binds to the active site of these enzymes, preventing them from catalyzing necessary reactions in the viral life cycle. This disruption leads to the inhibition of viral replication and the eventual clearance of the virus from the host.
Molecular Targets and Pathways
Viral Polymerases: this compound targets viral RNA or DNA polymerases, inhibiting the synthesis of viral genetic material.
Proteases: The compound also inhibits viral proteases, preventing the processing of viral polyproteins into functional proteins.
Entry Inhibitors: In some cases, this compound can block the entry of viruses into host cells by binding to viral surface proteins.
Comparison with Similar Compounds
Antiviral agent 23 is compared with other similar compounds to highlight its uniqueness:
Remdesivir: Both compounds inhibit viral RNA polymerases, but this compound has shown broader activity against multiple virus families.
Favipiravir: While both are nucleoside analogs, this compound has a different mechanism of action and targets additional viral enzymes.
Molnupiravir: Similar in structure, but this compound has demonstrated higher potency in preclinical studies.
List of Similar Compounds
- Remdesivir
- Favipiravir
- Molnupiravir
- Ribavirin
- Acyclovir
This compound stands out due to its broad-spectrum activity, unique mechanism of action, and potential for use in various scientific and medical applications. Further research and development are ongoing to fully realize its potential as a versatile antiviral agent.
Properties
Molecular Formula |
C18H21N5O4 |
---|---|
Molecular Weight |
371.4 g/mol |
IUPAC Name |
(2R,4S,5R)-2-(hydroxymethyl)-5-[6-[(3-methylphenyl)methylamino]purin-9-yl]oxolane-3,4-diol |
InChI |
InChI=1S/C18H21N5O4/c1-10-3-2-4-11(5-10)6-19-16-13-17(21-8-20-16)23(9-22-13)18-15(26)14(25)12(7-24)27-18/h2-5,8-9,12,14-15,18,24-26H,6-7H2,1H3,(H,19,20,21)/t12-,14?,15+,18-/m1/s1 |
InChI Key |
WBJVJFOITZTVRG-OWYXCUOISA-N |
Isomeric SMILES |
CC1=CC(=CC=C1)CNC2=C3C(=NC=N2)N(C=N3)[C@H]4[C@H](C([C@H](O4)CO)O)O |
Canonical SMILES |
CC1=CC(=CC=C1)CNC2=C3C(=NC=N2)N(C=N3)C4C(C(C(O4)CO)O)O |
Origin of Product |
United States |
Disclaimer and Information on In-Vitro Research Products
Please be aware that all articles and product information presented on BenchChem are intended solely for informational purposes. The products available for purchase on BenchChem are specifically designed for in-vitro studies, which are conducted outside of living organisms. In-vitro studies, derived from the Latin term "in glass," involve experiments performed in controlled laboratory settings using cells or tissues. It is important to note that these products are not categorized as medicines or drugs, and they have not received approval from the FDA for the prevention, treatment, or cure of any medical condition, ailment, or disease. We must emphasize that any form of bodily introduction of these products into humans or animals is strictly prohibited by law. It is essential to adhere to these guidelines to ensure compliance with legal and ethical standards in research and experimentation.