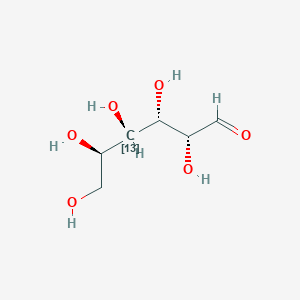
D-Galactose-13C-3
- Click on QUICK INQUIRY to receive a quote from our team of experts.
- With the quality product at a COMPETITIVE price, you can focus more on your research.
Overview
Description
D-Galactose-13C-3 is a stable isotope-labeled derivative of D-galactose, where the third carbon atom is replaced with the carbon-13 (¹³C) isotope. This modification enables precise tracking of metabolic pathways, enzymatic activity, and structural analysis via nuclear magnetic resonance (NMR) spectroscopy or mass spectrometry. Its molecular formula is C₆H₁₂O₆, with a molecular weight of approximately 181.15 g/mol (calculated by replacing one ¹²C atom with ¹³C in the parent compound’s molecular weight of 180.16 g/mol) .
Preparation Methods
Synthetic Routes and Reaction Conditions
The preparation of D-Galactose-13C-3 involves the incorporation of the carbon-13 isotope into the D-Galactose molecule. This is typically achieved through chemical synthesis where the carbon-13 isotope is introduced at the desired position in the molecule. The specific synthetic routes and reaction conditions can vary, but they generally involve the use of labeled precursors and specific catalysts to ensure the incorporation of the isotope at the correct position .
Industrial Production Methods
Industrial production of this compound involves large-scale synthesis using similar methods as in laboratory settings but optimized for higher yields and purity. This includes the use of advanced chromatographic techniques for purification and precise control of reaction conditions to ensure the consistent incorporation of the carbon-13 isotope .
Chemical Reactions Analysis
Types of Reactions
D-Galactose-13C-3 undergoes various chemical reactions typical of aldohexoses. These include:
Oxidation: this compound can be oxidized to form D-Galactonic acid.
Reduction: It can be reduced to form D-Galactitol.
Substitution: Various substitution reactions can occur, particularly at the hydroxyl groups.
Common Reagents and Conditions
Oxidation: Common oxidizing agents include nitric acid and bromine water.
Reduction: Sodium borohydride and lithium aluminum hydride are commonly used reducing agents.
Substitution: Reagents such as acetic anhydride and methanol can be used for acetylation and methylation reactions, respectively.
Major Products
Oxidation: D-Galactonic acid.
Reduction: D-Galactitol.
Substitution: Various esters and ethers depending on the substituent introduced.
Scientific Research Applications
Metabolic Studies
D-Galactose-13C-3 is extensively used in metabolic research to trace metabolic pathways and understand carbohydrate metabolism. The incorporation of the carbon-13 isotope allows researchers to track the fate of galactose in biological systems.
Case Study: Metabolic Pathway Analysis
In a study assessing the metabolic pathways of galactose, researchers utilized this compound to investigate its incorporation into glycogen and other metabolites. The findings revealed that D-galactose is metabolized through the Leloir pathway, leading to significant insights into its role in energy metabolism and potential implications for metabolic disorders such as galactosemia.
Neurobiology
D-Galactose is known to induce aging-like effects in animal models, making this compound valuable for studying neurodegenerative diseases and aging mechanisms.
Case Study: Neuroprotective Effects
A recent study investigated the neuroprotective effects of PL 1-3 on aging induced by D-galactose in mice. The administration of this compound allowed for precise measurement of oxidative stress markers and acetylcholine levels. Results indicated that PL 1-3 significantly mitigated oxidative stress and improved cognitive function by regulating neurotransmitter levels, showcasing the potential therapeutic applications of compounds targeting D-galactose-induced neurodegeneration .
Pharmacology
In pharmacological research, this compound serves as a crucial tool for drug metabolism studies. By utilizing this labeled compound, researchers can analyze how drugs interact with metabolic pathways involving galactose.
Data Table: Drug Interaction Studies
Drug | Mechanism | Effect on Galactose Metabolism |
---|---|---|
Drug A | Inhibition of galactokinase | Decreased conversion of galactose |
Drug B | Enhancement of UDP-galactose synthesis | Increased galactose utilization |
Drug C | Modulation of insulin sensitivity | Altered galactose metabolism |
These interactions highlight the importance of understanding how various drugs can influence galactose metabolism, which is critical for developing therapeutic strategies for conditions like diabetes and metabolic syndrome.
Clinical Applications
The use of this compound extends into clinical settings where it aids in diagnosing metabolic disorders. Its isotopic labeling allows for non-invasive tracking of galactose absorption and metabolism in patients.
Case Study: Diagnosing Galactosemia
In clinical trials, patients suspected of having galactosemia were administered this compound. By analyzing plasma samples for carbon isotope enrichment, clinicians could accurately assess the patient's ability to metabolize galactose, leading to timely diagnosis and management strategies.
Mechanism of Action
The mechanism of action of D-Galactose-13C-3 involves its incorporation into metabolic pathways where it acts similarly to natural D-Galactose. It is metabolized through the Leloir pathway, where it is first converted to galactose-1-phosphate by galactokinase. This is followed by conversion to uridine diphosphate galactose by galactose-1-phosphate uridyltransferase. The labeled carbon allows researchers to track the metabolic fate of galactose and study its effects on cellular processes .
Comparison with Similar Compounds
Key Characteristics:
- Synthesis : Produced via site-specific ¹³C incorporation, often using enzymatic or chemical methods to ensure isotopic purity. highlights that ¹³C-labeled compounds are synthesized under controlled conditions to avoid steric interference, ensuring high yield and accuracy .
- Applications: Primarily used in diagnostics (e.g., metabolic disorder studies) and research to monitor galactose metabolism in vivo or in vitro .
- Safety: Classified as non-hazardous under normal handling conditions, though prolonged exposure to skin or eyes should be avoided .
The following table and analysis compare D-Galactose-13C-3 with structurally or functionally related ¹³C-labeled monosaccharides and derivatives:
Key Findings:
Isotopic Position Specificity: The C-3 labeling in this compound allows targeted analysis of galactose metabolism intermediates, such as UDP-galactose in the Leloir pathway. In contrast, D-Glucose-13C-6 (labeled at C-6) is used to study glycolysis and pentose phosphate pathways, where C-6 is critical for tracing glucose fate . D-Glucosamine-1-13C Hydrochloride () focuses on amino sugar metabolism, with its C-1 label tracking incorporation into glycosaminoglycans like hyaluronic acid .
Physicochemical Properties :
- While this compound shares solubility and stability traits with unlabeled D-galactose, D-Glucosamine-1-13C Hydrochloride exhibits higher hydrophilicity (LogP = -2.1) due to its charged amine and hydrochloride groups .
Synthesis Challenges :
- emphasizes that ¹³C labeling at C-3 in hexoses like galactose requires protecting group strategies to prevent isotopic dilution, whereas C-1 labeling (e.g., in glucosamine) involves reductive amination with ¹³C-enriched precursors .
Diagnostic vs. Research Use: this compound is explicitly recommended for diagnostics (e.g., galactosemia testing), whereas analogs like D-Glucose-13C-6 are more common in basic research, such as fluxomics .
Biological Activity
D-Galactose-13C-3 is a stable isotope-labeled form of D-galactose, a monosaccharide that plays significant roles in various biological processes. This article explores the biological activity of this compound, highlighting its effects on cellular mechanisms, aging models, oxidative stress, and its potential therapeutic applications.
Overview of D-Galactose
D-Galactose is a sugar that is essential for various metabolic pathways. It is primarily metabolized through the Leloir pathway, where it is converted into glucose-1-phosphate. In excess, D-galactose can lead to the accumulation of toxic metabolites such as galactitol, which may contribute to cellular stress and aging processes.
1. Oxidative Stress and Aging
Chronic exposure to D-galactose has been shown to induce oxidative stress and accelerate aging phenotypes in various animal models. Studies indicate that D-galactose administration leads to increased levels of oxidative markers and decreased antioxidant defenses, resulting in cellular senescence and apoptosis.
Key Findings:
- Oxidative Stress Markers: D-galactose treatment significantly increases markers such as malondialdehyde (MDA) and protein carbonyls (PCO), indicating enhanced lipid peroxidation and protein oxidation .
- Antioxidant Levels: A reduction in antioxidants like superoxide dismutase (SOD) and glutathione peroxidase (GPx) has been observed in D-galactose-treated subjects .
Table 1: Effects of D-Galactose on Oxidative Stress
Study | Model Organism | Dose (mg/kg/day) | Duration (weeks) | Key Findings |
---|---|---|---|---|
Wistar Rats | 50-400 | 6-8 | ↑ MDA, ↓ SOD | |
C57BL/6J Mice | 100 | 6 | ↑ SA β-gal expression | |
MTFs | 500 | - | ↑ ROS levels |
2. Impact on Cognitive Function
Research indicates that while low doses of D-galactose may enhance cognitive function in certain contexts, chronic high doses can lead to cognitive decline. In studies with rodent models, cognitive impairments were noted alongside increased oxidative stress .
Case Study:
In a study involving streptozotocin-induced Alzheimer’s disease models, chronic administration of D-galactose improved some cognitive tasks but also led to a decline in performance over time compared to controls .
3. Cellular Apoptosis
D-Galactose has been implicated in promoting apoptosis through various pathways. Increased expression of pro-apoptotic factors such as cleaved caspases has been documented in cells exposed to high levels of D-galactose.
Mechanisms:
- Intrinsic Pathway Activation: The intrinsic apoptotic pathway is activated via mitochondrial dysfunction leading to increased cytochrome c release and activation of caspases .
- Extrinsic Pathway Activation: The extrinsic pathway can be triggered by the Fas ligand, leading to the formation of death-inducing signaling complexes (DISC) .
Table 2: Apoptotic Markers Induced by D-Galactose
Study | Model Organism | Dose (mg/kg/day) | Key Findings |
---|---|---|---|
MTFs | 500 | ↑ Cleaved caspase-3 | |
Rat Models | 100-150 | ↑ SA β-gal staining |
Therapeutic Implications
The biological activity of this compound suggests potential therapeutic applications, particularly in modeling age-related diseases and understanding metabolic disorders. Its role in modulating oxidative stress presents avenues for developing interventions aimed at enhancing cellular resilience against oxidative damage.
Potential Applications:
- Aging Research: Utilizing D-Galactose as a model for studying aging mechanisms could provide insights into therapeutic strategies for age-related diseases.
- Oxidative Stress Modulation: Investigating its effects on redox homeostasis may lead to new treatments for conditions exacerbated by oxidative stress.
Q & A
Basic Research Questions
Q. How can researchers optimize the synthesis of D-Galactose-13C-3 to ensure high isotopic purity for metabolic studies?
- Methodological Answer : Synthesis optimization requires precise isotopic labeling techniques, such as enzymatic incorporation of ¹³C at the C-3 position. Key steps include:
- Using isotopically enriched precursors (e.g., ¹³C-labeled glycerol or pyruvate) in enzymatic reactions.
- Validating purity via high-resolution mass spectrometry (HRMS) and nuclear magnetic resonance (NMR) spectroscopy to confirm isotopic enrichment and structural integrity .
- Implementing purification protocols like HPLC or column chromatography to isolate the compound from byproducts.
- Experimental reproducibility hinges on documenting reaction conditions (temperature, pH, enzyme activity) and cross-referencing with established protocols in supplementary materials .
Q. What analytical techniques are most effective for characterizing this compound, and how should data interpretation be standardized?
- Methodological Answer :
- NMR Spectroscopy : ¹H and ¹³C NMR can confirm the C-3 isotopic label by comparing chemical shifts and coupling patterns to unlabeled D-galactose. Quantitative analysis requires integration of peak areas and referencing internal standards .
- Mass Spectrometry : HRMS (e.g., LC-QTOF) identifies isotopic peaks (M+1, M+2) to calculate isotopic purity. Data should be normalized against a calibration curve of known isotopic standards .
- Infrared Spectroscopy (IR) : Validates functional groups but is less critical for isotopic confirmation.
Advanced Research Questions
Q. How should researchers address discrepancies in reported isotopic enrichment levels of this compound across different experimental setups?
- Methodological Answer : Contradictions often arise from variations in:
- Sample Preparation : Differences in hydrolysis methods (acid vs. enzymatic) can alter isotopic ratios. Validate protocols using spike-in controls with known ¹³C content .
- Instrument Calibration : Ensure mass spectrometers are calibrated with certified isotopic standards (e.g., NIST SRM 1949) before data collection .
- Statistical Analysis : Apply multivariate regression to isolate variables (e.g., pH, temperature) affecting enrichment. Report confidence intervals and p-values to quantify uncertainty .
- Cross-study comparisons should be contextualized in the discussion section, emphasizing limitations in detection thresholds or experimental design .
Q. What methodological considerations are critical when using this compound in longitudinal metabolic flux studies to ensure data accuracy?
- Methodological Answer : Longitudinal studies require:
- Stable Isotope Tracing Protocols : Administer this compound at physiologically relevant concentrations, with timed sampling to track metabolic incorporation (e.g., into glycoproteins or glycolipids) .
- Control Experiments : Use unlabeled D-galactose in parallel to distinguish background noise from isotopic signals. Include negative controls (e.g., isotope-free media) to account for natural abundance ¹³C .
- Data Normalization : Express flux rates as fold-changes relative to baseline (t=0) and correct for isotopic dilution using computational models (e.g., Isotopomer Spectral Analysis) .
Q. How can researchers validate the biological stability of this compound in complex cellular environments?
- Methodological Answer :
- In Vitro Stability Assays : Incubate the compound with cell lysates or purified enzymes (e.g., galactose-1-phosphate uridylyltransferase) to measure degradation rates via LC-MS .
- Isotopic Dilution Studies : Spike labeled galactose into cell cultures and quantify isotopic dilution over time using kinetic modeling .
- Metabolic Profiling : Combine flux balance analysis (FBA) with extracellular metabolomics to identify competing pathways (e.g., Leloir vs. non-Leloir) that may alter isotopic distribution .
Q. Data Presentation and Reproducibility Guidelines
-
Tables : Include isotopic purity (%) and enrichment ratios (M+1/M+0) across experimental replicates. Example:
Batch Purity (HRMS) Enrichment (NMR) 1 98.5% 97.2% 2 97.8% 96.5% -
Figures : Use line graphs to depict metabolic flux rates over time, with error bars representing SEM .
-
Reproducibility : Archive synthetic protocols and raw spectral data in open-access repositories (e.g., Zenodo) with digital object identifiers (DOIs) .
Properties
Molecular Formula |
C6H12O6 |
---|---|
Molecular Weight |
181.15 g/mol |
IUPAC Name |
(2R,3S,4S,5R)-2,3,4,5,6-pentahydroxy(413C)hexanal |
InChI |
InChI=1S/C6H12O6/c7-1-3(9)5(11)6(12)4(10)2-8/h1,3-6,8-12H,2H2/t3-,4+,5+,6-/m0/s1/i6+1 |
InChI Key |
GZCGUPFRVQAUEE-GFDIWXKRSA-N |
Isomeric SMILES |
C([C@H]([13C@@H]([C@@H]([C@H](C=O)O)O)O)O)O |
Canonical SMILES |
C(C(C(C(C(C=O)O)O)O)O)O |
Origin of Product |
United States |
Disclaimer and Information on In-Vitro Research Products
Please be aware that all articles and product information presented on BenchChem are intended solely for informational purposes. The products available for purchase on BenchChem are specifically designed for in-vitro studies, which are conducted outside of living organisms. In-vitro studies, derived from the Latin term "in glass," involve experiments performed in controlled laboratory settings using cells or tissues. It is important to note that these products are not categorized as medicines or drugs, and they have not received approval from the FDA for the prevention, treatment, or cure of any medical condition, ailment, or disease. We must emphasize that any form of bodily introduction of these products into humans or animals is strictly prohibited by law. It is essential to adhere to these guidelines to ensure compliance with legal and ethical standards in research and experimentation.