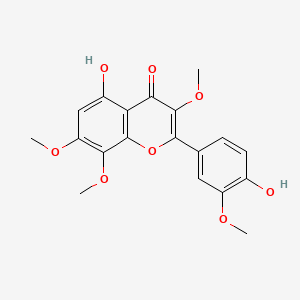
4',5-Dihydroxy-3,3',7,8-tetramethoxyflavone
Overview
Description
4',5-Dihydroxy-3,3',7,8-tetramethoxyflavone is a naturally occurring cyclic peptide produced by several species of fungi. It has garnered significant interest due to its cytotoxic and anti-adipogenic properties. This compound is known for its ability to kill mammalian cells at high doses, making it a promising candidate for cancer treatment research .
Mechanism of Action
Target of Action
Ternatin, a fungus-born poison, primarily targets the translation elongation factor 1A (eEF1A) . eEF1A is a key player in protein translation, participating in a series of reactions with guanine nucleotides, transfer RNAs (tRNAs), a guanine nucleotide exchange factor, and the elongating ribosome . It forms a ternary complex with GTP and aminoacyl-tRNA (aa-tRNA), which is crucial for protein synthesis .
Mode of Action
Ternatin disrupts protein translation by binding to eEF1A . Specifically, it binds to the eEF1A ternary complex with GTP and aa-tRNA . This interaction traps eEF1A on elongating ribosomes, inhibiting the translation process . Mutations in domain III of eEF1A can prevent ternatin binding and confer resistance to its cytotoxic effects .
Biochemical Pathways
The primary biochemical pathway affected by ternatin is protein translation . By binding to eEF1A, ternatin disrupts the carefully orchestrated series of reactions that eEF1A participates in, including interactions with guanine nucleotides, tRNAs, a guanine nucleotide exchange factor, and the elongating ribosome . This disruption leads to the inhibition of protein synthesis .
Pharmacokinetics
These properties play a crucial role in determining the bioavailability of a compound
Result of Action
The binding of ternatin to the eEF1A ternary complex results in the disruption of protein translation . This disruption leads to the inhibition of protein synthesis, which can have cytotoxic effects . Ternatin and its synthetic variants have been found to be cytotoxic towards cancer cells, with some variants exhibiting up to 500-fold greater potency than ternatin itself .
Action Environment
Environmental factors can significantly impact the effectiveness of many compounds
Biochemical Analysis
Biochemical Properties
Ternatin plays a crucial role in inhibiting protein translation by binding to the translation elongation factor 1A (eEF1A). This interaction disrupts the normal function of eEF1A, which is essential for the elongation phase of protein synthesis. Ternatin specifically binds to the eEF1A·GTP·aminoacyl-tRNA complex, preventing the proper accommodation of aminoacyl-tRNA into the ribosome . This inhibition leads to a halt in protein synthesis, which is particularly detrimental to rapidly dividing cells, such as cancer cells.
Cellular Effects
Ternatin exerts profound effects on various cell types and cellular processes. In cancer cells, Ternatin induces cytotoxicity by disrupting protein synthesis, leading to cell death . It also affects adipocytes by inhibiting adipogenesis at low nanomolar concentrations . Ternatin’s impact on cell signaling pathways includes the inhibition of the elongation phase of protein synthesis, which can lead to altered gene expression and cellular metabolism. This compound’s ability to interfere with protein translation makes it a potent anti-cancer agent.
Molecular Mechanism
The molecular mechanism of Ternatin involves its binding to the translation elongation factor 1A (eEF1A) complex. By binding to eEF1A, Ternatin prevents the release of aminoacyl-tRNA, thereby stalling the ribosome during translation elongation . This stalling triggers quality control pathways that lead to the degradation of eEF1A through the ubiquitin-proteasome system . The degradation of eEF1A further exacerbates the inhibition of protein synthesis, contributing to Ternatin’s cytotoxic effects.
Temporal Effects in Laboratory Settings
In laboratory settings, the effects of Ternatin have been observed to change over time. Ternatin-4, a synthetic variant of Ternatin, has shown reversible effects upon washout, whereas other related compounds exhibit irreversible effects . The stability and degradation of Ternatin in vitro and in vivo are critical factors that influence its long-term effects on cellular function. Studies have shown that Ternatin can induce degradation of eEF1A, which is specific to its mechanism of action .
Dosage Effects in Animal Models
The effects of Ternatin vary with different dosages in animal models. At low nanomolar concentrations, Ternatin inhibits adipogenesis, while higher concentrations induce cytotoxicity . In animal studies, Ternatin has demonstrated a dose-dependent response, with higher doses leading to increased toxicity. The threshold effects and potential adverse effects at high doses are important considerations for its therapeutic application.
Metabolic Pathways
Ternatin is involved in metabolic pathways related to protein synthesis. By inhibiting the translation elongation factor 1A (eEF1A), Ternatin disrupts the normal flow of protein synthesis, leading to changes in metabolic flux and metabolite levels . The interaction of Ternatin with eEF1A and its impact on the elongation phase of protein synthesis are central to its metabolic effects.
Transport and Distribution
Within cells and tissues, Ternatin is transported and distributed through interactions with specific transporters and binding proteins. The localization and accumulation of Ternatin are influenced by its binding to the eEF1A complex . The transport and distribution of Ternatin are critical for its efficacy and potency as a therapeutic agent.
Subcellular Localization
Ternatin’s subcellular localization is primarily associated with its binding to the translation elongation factor 1A (eEF1A) complex. This localization is essential for its activity and function in inhibiting protein synthesis . The targeting signals and post-translational modifications that direct Ternatin to specific compartments or organelles are crucial for its mechanism of action.
Preparation Methods
Synthetic Routes and Reaction Conditions
4',5-Dihydroxy-3,3',7,8-tetramethoxyflavone can be synthesized through a series of chemical reactions involving the formation of peptide bonds. The synthesis typically starts with the assembly of the linear peptide chain, followed by cyclization to form the cyclic structure. The reaction conditions often involve the use of coupling reagents such as dicyclohexylcarbodiimide (DCC) and N-hydroxysuccinimide (NHS) to facilitate peptide bond formation .
Industrial Production Methods
Industrial production of ternatin involves fermentation processes using fungi that naturally produce the compound. The fungi are cultured in large bioreactors under controlled conditions to maximize the yield of ternatin. The compound is then extracted and purified using techniques such as high-performance liquid chromatography (HPLC) .
Chemical Reactions Analysis
Types of Reactions
4',5-Dihydroxy-3,3',7,8-tetramethoxyflavone undergoes various chemical reactions, including oxidation, reduction, and substitution. These reactions are essential for modifying the compound to enhance its biological activity and stability .
Common Reagents and Conditions
Oxidation: Common oxidizing agents such as hydrogen peroxide (H₂O₂) and potassium permanganate (KMnO₄) are used to introduce oxygen-containing functional groups into ternatin.
Reduction: Reducing agents like sodium borohydride (NaBH₄) and lithium aluminum hydride (LiAlH₄) are employed to reduce specific functional groups within the molecule.
Major Products Formed
The major products formed from these reactions include various derivatives of ternatin with altered functional groups. These derivatives are often tested for their enhanced biological activity and potential therapeutic applications .
Scientific Research Applications
4',5-Dihydroxy-3,3',7,8-tetramethoxyflavone has a wide range of scientific research applications:
Chemistry: this compound is used as a chemical probe to study protein synthesis and translation elongation processes.
Biology: It serves as a tool to investigate the mechanisms of cell death and protein synthesis inhibition.
Medicine: this compound and its synthetic variants are being explored for their potential as cancer therapeutics due to their ability to target the elongation factor-1A ternary complex.
Industry: This compound is used in the development of new drugs and as a model compound for studying cyclic peptides
Comparison with Similar Compounds
4',5-Dihydroxy-3,3',7,8-tetramethoxyflavone is often compared with other cyclic peptides such as didemnin and cytotrienin. These compounds share similar mechanisms of action by targeting the eEF1A ternary complex. ternatin exhibits unique properties, such as its specific binding site on eEF1A and its ability to induce eEF1A degradation. This makes ternatin a valuable tool for studying protein synthesis and developing new cancer therapeutics .
List of Similar Compounds
- Didemnin
- Cytotrienin
- Cycloheximide
- Anisomycin
Properties
IUPAC Name |
5-hydroxy-2-(4-hydroxy-3-methoxyphenyl)-3,7,8-trimethoxychromen-4-one | |
---|---|---|
Source | PubChem | |
URL | https://pubchem.ncbi.nlm.nih.gov | |
Description | Data deposited in or computed by PubChem | |
InChI |
InChI=1S/C19H18O8/c1-23-12-7-9(5-6-10(12)20)16-19(26-4)15(22)14-11(21)8-13(24-2)17(25-3)18(14)27-16/h5-8,20-21H,1-4H3 | |
Source | PubChem | |
URL | https://pubchem.ncbi.nlm.nih.gov | |
Description | Data deposited in or computed by PubChem | |
InChI Key |
DGUWNYKZOJRCQQ-UHFFFAOYSA-N | |
Source | PubChem | |
URL | https://pubchem.ncbi.nlm.nih.gov | |
Description | Data deposited in or computed by PubChem | |
Canonical SMILES |
COC1=C(C=CC(=C1)C2=C(C(=O)C3=C(O2)C(=C(C=C3O)OC)OC)OC)O | |
Source | PubChem | |
URL | https://pubchem.ncbi.nlm.nih.gov | |
Description | Data deposited in or computed by PubChem | |
Molecular Formula |
C19H18O8 | |
Source | PubChem | |
URL | https://pubchem.ncbi.nlm.nih.gov | |
Description | Data deposited in or computed by PubChem | |
DSSTOX Substance ID |
DTXSID40205714 | |
Record name | Ternatin (flavonoid) | |
Source | EPA DSSTox | |
URL | https://comptox.epa.gov/dashboard/DTXSID40205714 | |
Description | DSSTox provides a high quality public chemistry resource for supporting improved predictive toxicology. | |
Molecular Weight |
374.3 g/mol | |
Source | PubChem | |
URL | https://pubchem.ncbi.nlm.nih.gov | |
Description | Data deposited in or computed by PubChem | |
CAS No. |
571-71-1 | |
Record name | Ternatin (flavonoid) | |
Source | ChemIDplus | |
URL | https://pubchem.ncbi.nlm.nih.gov/substance/?source=chemidplus&sourceid=0000571711 | |
Description | ChemIDplus is a free, web search system that provides access to the structure and nomenclature authority files used for the identification of chemical substances cited in National Library of Medicine (NLM) databases, including the TOXNET system. | |
Record name | Ternatin (flavonoid) | |
Source | EPA DSSTox | |
URL | https://comptox.epa.gov/dashboard/DTXSID40205714 | |
Description | DSSTox provides a high quality public chemistry resource for supporting improved predictive toxicology. | |
Record name | 5,4'-DIHYDROXY-3,7,8,3'-TETRAMETHOXYFLAVONE | |
Source | FDA Global Substance Registration System (GSRS) | |
URL | https://gsrs.ncats.nih.gov/ginas/app/beta/substances/G60G0VQS3I | |
Description | The FDA Global Substance Registration System (GSRS) enables the efficient and accurate exchange of information on what substances are in regulated products. Instead of relying on names, which vary across regulatory domains, countries, and regions, the GSRS knowledge base makes it possible for substances to be defined by standardized, scientific descriptions. | |
Explanation | Unless otherwise noted, the contents of the FDA website (www.fda.gov), both text and graphics, are not copyrighted. They are in the public domain and may be republished, reprinted and otherwise used freely by anyone without the need to obtain permission from FDA. Credit to the U.S. Food and Drug Administration as the source is appreciated but not required. | |
Retrosynthesis Analysis
AI-Powered Synthesis Planning: Our tool employs the Template_relevance Pistachio, Template_relevance Bkms_metabolic, Template_relevance Pistachio_ringbreaker, Template_relevance Reaxys, Template_relevance Reaxys_biocatalysis model, leveraging a vast database of chemical reactions to predict feasible synthetic routes.
One-Step Synthesis Focus: Specifically designed for one-step synthesis, it provides concise and direct routes for your target compounds, streamlining the synthesis process.
Accurate Predictions: Utilizing the extensive PISTACHIO, BKMS_METABOLIC, PISTACHIO_RINGBREAKER, REAXYS, REAXYS_BIOCATALYSIS database, our tool offers high-accuracy predictions, reflecting the latest in chemical research and data.
Strategy Settings
Precursor scoring | Relevance Heuristic |
---|---|
Min. plausibility | 0.01 |
Model | Template_relevance |
Template Set | Pistachio/Bkms_metabolic/Pistachio_ringbreaker/Reaxys/Reaxys_biocatalysis |
Top-N result to add to graph | 6 |
Feasible Synthetic Routes
Disclaimer and Information on In-Vitro Research Products
Please be aware that all articles and product information presented on BenchChem are intended solely for informational purposes. The products available for purchase on BenchChem are specifically designed for in-vitro studies, which are conducted outside of living organisms. In-vitro studies, derived from the Latin term "in glass," involve experiments performed in controlled laboratory settings using cells or tissues. It is important to note that these products are not categorized as medicines or drugs, and they have not received approval from the FDA for the prevention, treatment, or cure of any medical condition, ailment, or disease. We must emphasize that any form of bodily introduction of these products into humans or animals is strictly prohibited by law. It is essential to adhere to these guidelines to ensure compliance with legal and ethical standards in research and experimentation.