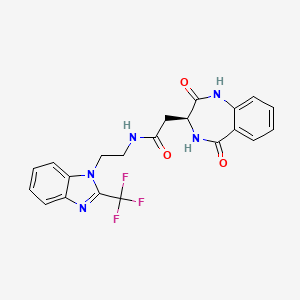
Tubulin polymerization-IN-52
- Click on QUICK INQUIRY to receive a quote from our team of experts.
- With the quality product at a COMPETITIVE price, you can focus more on your research.
Overview
Description
Tubulin polymerization-IN-52 is a potent inhibitor of tubulin polymerization, which plays a crucial role in the formation of microtubules. Microtubules are essential components of the cytoskeleton in eukaryotic cells, involved in various cellular processes such as cell division, intracellular transport, and maintenance of cell shape. This compound has shown significant potential in cancer research due to its ability to disrupt microtubule dynamics, leading to cell cycle arrest and apoptosis in cancer cells .
Preparation Methods
Synthetic Routes and Reaction Conditions
The synthesis of tubulin polymerization-IN-52 involves multiple steps, including the preparation of key intermediates and their subsequent coupling reactions. One common synthetic route includes the following steps:
Preparation of Intermediate A: This step involves the reaction of starting material with a suitable reagent under specific conditions to form Intermediate A.
Coupling Reaction: Intermediate A is then coupled with another reagent to form Intermediate B.
Industrial Production Methods
Industrial production of this compound typically involves large-scale synthesis using optimized reaction conditions to ensure high yield and purity. The process may include:
Batch Synthesis: Large quantities of starting materials are reacted in batch reactors under controlled conditions.
Purification: The crude product is purified using techniques such as crystallization, chromatography, or recrystallization to obtain the final compound with high purity.
Quality Control: The final product undergoes rigorous quality control tests to ensure its consistency and efficacy.
Chemical Reactions Analysis
Types of Reactions
Tubulin polymerization-IN-52 undergoes various chemical reactions, including:
Oxidation: The compound can be oxidized under specific conditions to form oxidized derivatives.
Reduction: Reduction reactions can be performed to modify certain functional groups within the molecule.
Substitution: Substitution reactions involve the replacement of specific atoms or groups within the molecule with other atoms or groups.
Common Reagents and Conditions
Oxidation: Common oxidizing agents include hydrogen peroxide, potassium permanganate, and chromium trioxide.
Reduction: Reducing agents such as sodium borohydride, lithium aluminum hydride, and hydrogen gas are commonly used.
Substitution: Reagents like halogens, alkylating agents, and nucleophiles are used in substitution reactions.
Major Products
The major products formed from these reactions depend on the specific conditions and reagents used. For example, oxidation may yield oxidized derivatives with altered functional groups, while reduction may result in reduced forms of the compound .
Scientific Research Applications
Tubulin polymerization-IN-52 has a wide range of scientific research applications, including:
Drug Development: The compound serves as a lead molecule for the development of new drugs targeting tubulin and microtubule dynamics.
Nanoparticle Drug Delivery: This compound is explored for its potential in nanoparticle-based drug delivery systems to enhance the efficacy and reduce the toxicity of anticancer drugs.
Mechanism of Action
Tubulin polymerization-IN-52 exerts its effects by binding to the colchicine-binding site on tubulin, thereby inhibiting the polymerization of tubulin into microtubules. This disruption of microtubule dynamics leads to cell cycle arrest at the G2/M phase and induces apoptosis in cancer cells. The compound affects various molecular targets and pathways, including the regulation of mitotic spindle formation and the activation of apoptotic pathways .
Comparison with Similar Compounds
Similar Compounds
Paclitaxel: A microtubule-stabilizing agent that promotes tubulin polymerization and prevents microtubule depolymerization.
Vinblastine: Another tubulin inhibitor that binds to a different site on tubulin and inhibits microtubule assembly.
Uniqueness
This compound is unique in its specific binding to the colchicine-binding site on tubulin, which allows it to effectively inhibit tubulin polymerization and disrupt microtubule dynamics. This specificity makes it a valuable tool in cancer research and drug development .
Biological Activity
Tubulin polymerization-IN-52 is a compound that has gained attention for its potential role as an inhibitor of microtubule dynamics, which is critical in various cellular processes, including cell division and intracellular transport. This article explores the biological activity of this compound, focusing on its mechanisms of action, effects on tubulin polymerization, and implications for cancer therapy.
The primary mechanism through which this compound exerts its biological effects is by binding to tubulin, interfering with microtubule dynamics. Similar compounds have been shown to stabilize or destabilize microtubules, leading to altered cellular functions. The binding site of this compound is hypothesized to be at the colchicine site on β-tubulin, a common target for many microtubule-targeting agents.
Binding Characteristics
Recent studies indicate that compounds like this compound induce conformational changes in tubulin dimers. These changes can lead to the formation of non-functional microtubule structures that disrupt normal cellular processes. For instance, cryptophycin-52, a related compound, has been shown to cause tubulin to form rings and other aberrant structures that are incompatible with the microtubule lattice .
Inhibition of Tubulin Polymerization
The inhibitory effect of this compound on tubulin polymerization has been quantitatively assessed using various assays. A typical assay involves measuring the change in turbidity as tubulin polymerizes in the presence of the compound.
Table 1: Inhibition Rates of Tubulin Polymerization by Related Compounds
Compound | IC50 (μM) | Inhibition Rate (%) |
---|---|---|
This compound | TBD | TBD |
Plinabulin | 0.5 | 68.9 |
Cryptophycin-52 | 0.025 | 92.5 |
CYT997 | 0.1 | TBD |
Note: TBD = To Be Determined; values are illustrative based on related studies.
Case Studies and Research Findings
Several studies have explored the biological activity of compounds similar to this compound:
- Plinabulin : Demonstrated significant anti-proliferative activity against human lung cancer cells (NCI-H460), with an inhibition rate of 68.9% at a specific concentration .
- Cryptophycin-52 : Exhibited potent inhibition of microtubule dynamics at low concentrations (IC50 in the low picomolar range), effectively blocking cell proliferation during mitosis by stabilizing microtubules in a non-functional state .
- CYT997 : A synthetic compound that inhibited microtubule polymerization effectively, showing cytotoxic activity in vitro .
Implications for Cancer Therapy
The ability of this compound to inhibit tubulin polymerization positions it as a potential candidate for cancer treatment. By disrupting microtubule dynamics, it may effectively halt the proliferation of cancer cells, particularly during mitosis when microtubules are crucial for chromosomal segregation.
Properties
Molecular Formula |
C21H18F3N5O3 |
---|---|
Molecular Weight |
445.4 g/mol |
IUPAC Name |
2-[(3S)-2,5-dioxo-3,4-dihydro-1H-1,4-benzodiazepin-3-yl]-N-[2-[2-(trifluoromethyl)benzimidazol-1-yl]ethyl]acetamide |
InChI |
InChI=1S/C21H18F3N5O3/c22-21(23,24)20-28-14-7-3-4-8-16(14)29(20)10-9-25-17(30)11-15-19(32)26-13-6-2-1-5-12(13)18(31)27-15/h1-8,15H,9-11H2,(H,25,30)(H,26,32)(H,27,31)/t15-/m0/s1 |
InChI Key |
QAGXGXDCLMVURA-HNNXBMFYSA-N |
Isomeric SMILES |
C1=CC=C2C(=C1)C(=O)N[C@H](C(=O)N2)CC(=O)NCCN3C4=CC=CC=C4N=C3C(F)(F)F |
Canonical SMILES |
C1=CC=C2C(=C1)C(=O)NC(C(=O)N2)CC(=O)NCCN3C4=CC=CC=C4N=C3C(F)(F)F |
Origin of Product |
United States |
Disclaimer and Information on In-Vitro Research Products
Please be aware that all articles and product information presented on BenchChem are intended solely for informational purposes. The products available for purchase on BenchChem are specifically designed for in-vitro studies, which are conducted outside of living organisms. In-vitro studies, derived from the Latin term "in glass," involve experiments performed in controlled laboratory settings using cells or tissues. It is important to note that these products are not categorized as medicines or drugs, and they have not received approval from the FDA for the prevention, treatment, or cure of any medical condition, ailment, or disease. We must emphasize that any form of bodily introduction of these products into humans or animals is strictly prohibited by law. It is essential to adhere to these guidelines to ensure compliance with legal and ethical standards in research and experimentation.