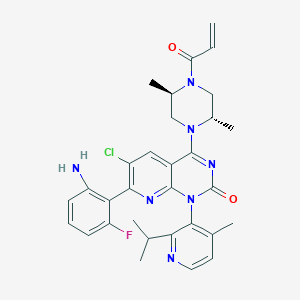
KRAS G12C inhibitor 61
- Click on QUICK INQUIRY to receive a quote from our team of experts.
- With the quality product at a COMPETITIVE price, you can focus more on your research.
Overview
Description
KRAS G12C inhibitor 61 is a small molecule compound specifically designed to target the KRAS G12C mutation, a common oncogenic driver in various cancers, including non-small cell lung cancer, colorectal cancer, and pancreatic cancer . This mutation results in the substitution of glycine with cysteine at the 12th position of the KRAS protein, leading to its constitutive activation and promoting uncontrolled cell proliferation .
Preparation Methods
Synthetic Routes and Reaction Conditions
The synthesis of KRAS G12C inhibitor 61 involves multiple steps, starting with the preparation of key intermediates. Common reaction conditions involve the use of organic solvents, catalysts, and temperature control to ensure high yield and purity .
Industrial Production Methods
Industrial production of this compound requires scaling up the laboratory synthesis while maintaining the quality and consistency of the product. This involves optimizing reaction conditions, using high-throughput reactors, and implementing stringent quality control measures . The process also includes purification steps such as crystallization and chromatography to obtain the final product in its pure form .
Chemical Reactions Analysis
Types of Reactions
KRAS G12C inhibitor 61 undergoes various chemical reactions, including:
Covalent Bond Formation: The compound forms a covalent bond with the cysteine residue at position 12 of the KRAS protein, effectively inhibiting its activity.
Substitution Reactions:
Common Reagents and Conditions
Major Products
The major product of these reactions is the this compound itself, characterized by its high binding affinity and specificity for the KRAS G12C mutant .
Scientific Research Applications
KRAS G12C inhibitor 61 has a wide range of scientific research applications, including:
Mechanism of Action
KRAS G12C inhibitor 61 exerts its effects by covalently binding to the cysteine residue at position 12 of the KRAS protein, locking it in an inactive GDP-bound state . This prevents the activation of downstream signaling pathways such as the RAF-MEK-ERK and PI3K-AKT-mTOR pathways, which are crucial for cell proliferation and survival . By inhibiting these pathways, the compound effectively suppresses tumor growth and progression .
Comparison with Similar Compounds
KRAS G12C inhibitor 61 is compared with other similar compounds, such as:
Sotorasib: Another KRAS G12C inhibitor that has shown efficacy in clinical trials but may face resistance mechanisms.
Adagrasib: Similar to sotorasib, it targets the KRAS G12C mutation but has different pharmacokinetic properties.
MRTX849: A KRAS G12C inhibitor with a distinct binding mode and efficacy profile.
Uniqueness
This compound is unique due to its specific binding affinity and selectivity for the KRAS G12C mutant, making it a promising candidate for targeted cancer therapy . Its ability to form a covalent bond with the mutant protein distinguishes it from other non-covalent inhibitors .
List of Similar Compounds
- Sotorasib
- Adagrasib
- MRTX849
- JNJ-74699157
- GDC-6036
Properties
Molecular Formula |
C31H33ClFN7O2 |
---|---|
Molecular Weight |
590.1 g/mol |
IUPAC Name |
7-(2-amino-6-fluorophenyl)-6-chloro-4-[(2S,5R)-2,5-dimethyl-4-prop-2-enoylpiperazin-1-yl]-1-(4-methyl-2-propan-2-ylpyridin-3-yl)pyrido[2,3-d]pyrimidin-2-one |
InChI |
InChI=1S/C31H33ClFN7O2/c1-7-24(41)38-14-19(6)39(15-18(38)5)29-20-13-21(32)27(25-22(33)9-8-10-23(25)34)36-30(20)40(31(42)37-29)28-17(4)11-12-35-26(28)16(2)3/h7-13,16,18-19H,1,14-15,34H2,2-6H3/t18-,19+/m1/s1 |
InChI Key |
HBHOIHAXRLHFTR-MOPGFXCFSA-N |
Isomeric SMILES |
C[C@@H]1CN([C@H](CN1C(=O)C=C)C)C2=NC(=O)N(C3=NC(=C(C=C32)Cl)C4=C(C=CC=C4F)N)C5=C(C=CN=C5C(C)C)C |
Canonical SMILES |
CC1CN(C(CN1C(=O)C=C)C)C2=NC(=O)N(C3=NC(=C(C=C32)Cl)C4=C(C=CC=C4F)N)C5=C(C=CN=C5C(C)C)C |
Origin of Product |
United States |
Disclaimer and Information on In-Vitro Research Products
Please be aware that all articles and product information presented on BenchChem are intended solely for informational purposes. The products available for purchase on BenchChem are specifically designed for in-vitro studies, which are conducted outside of living organisms. In-vitro studies, derived from the Latin term "in glass," involve experiments performed in controlled laboratory settings using cells or tissues. It is important to note that these products are not categorized as medicines or drugs, and they have not received approval from the FDA for the prevention, treatment, or cure of any medical condition, ailment, or disease. We must emphasize that any form of bodily introduction of these products into humans or animals is strictly prohibited by law. It is essential to adhere to these guidelines to ensure compliance with legal and ethical standards in research and experimentation.