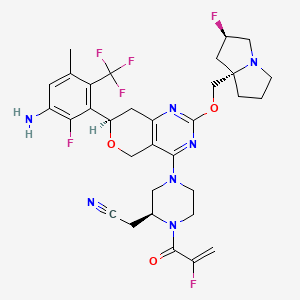
Krasg12C IN-2
- Click on QUICK INQUIRY to receive a quote from our team of experts.
- With the quality product at a COMPETITIVE price, you can focus more on your research.
Overview
Description
KRASG12C IN-2 is a compound that targets the KRAS G12C mutation, a common genetic alteration in various cancers, particularly non-small cell lung cancer (NSCLC). This mutation results in the substitution of glycine with cysteine at codon 12 of the KRAS gene, leading to the constitutive activation of the KRAS protein, which promotes uncontrolled cell proliferation and survival .
Preparation Methods
Synthetic Routes and Reaction Conditions: The synthesis of KRASG12C IN-2 involves multiple steps, starting with the preparation of key intermediates. The synthetic route typically includes the formation of a covalent bond with the thiol group of cysteine in the KRAS G12C mutant. This process often requires specific reaction conditions, such as controlled temperature and pH, to ensure the selective binding of the inhibitor to the target site .
Industrial Production Methods: Industrial production of this compound involves scaling up the laboratory synthesis process. This includes optimizing reaction conditions to maximize yield and purity while minimizing by-products. Advanced techniques such as high-performance liquid chromatography (HPLC) and mass spectrometry are used to monitor the production process and ensure the quality of the final product .
Chemical Reactions Analysis
Types of Reactions: KRASG12C IN-2 primarily undergoes covalent binding reactions with the thiol group of cysteine in the KRAS G12C mutant. This covalent modification traps KRAS in an inactive GDP-bound state, preventing its activation and subsequent signaling .
Common Reagents and Conditions: Common reagents used in the synthesis and reactions of this compound include organic solvents, reducing agents, and catalysts that facilitate the formation of covalent bonds. Reaction conditions such as temperature, pH, and solvent choice are critical to achieving the desired selectivity and efficiency .
Major Products Formed: The major product formed from the reaction of this compound with the KRAS G12C mutant is a covalently modified KRAS protein that is locked in an inactive state. This modification effectively inhibits the downstream signaling pathways that drive cancer cell proliferation and survival .
Scientific Research Applications
KRASG12C IN-2 has significant applications in scientific research, particularly in the fields of cancer biology and drug development. It is used to study the mechanisms of KRAS-driven cancers and to develop targeted therapies for patients with KRAS G12C mutations. The compound has shown promise in preclinical models of NSCLC, colorectal cancer, and pancreatic cancer, where it effectively inhibits tumor growth and enhances the efficacy of combination therapies .
Mechanism of Action
KRASG12C IN-2 exerts its effects by covalently binding to the thiol group of cysteine at codon 12 in the KRAS G12C mutant. This binding locks KRAS in an inactive GDP-bound state, preventing its activation and subsequent signaling through pathways such as RAF-MEK-ERK and PI3K-AKT-mTOR. By inhibiting these pathways, this compound effectively halts cancer cell proliferation and survival .
Comparison with Similar Compounds
KRASG12C IN-2 is unique among KRAS inhibitors due to its high selectivity for the G12C mutation and its ability to covalently modify the target protein. Similar compounds include sotorasib (AMG510) and adagrasib (MRTX849), which also target the KRAS G12C mutation but may differ in their binding affinities, pharmacokinetics, and clinical efficacy .
List of Similar Compounds:- Sotorasib (AMG510)
- Adagrasib (MRTX849)
- ARS-1620
- GDC-6036
This compound stands out due to its unique binding mechanism and potential for use in combination therapies to overcome resistance mechanisms in KRAS-driven cancers .
Properties
Molecular Formula |
C32H35F6N7O3 |
---|---|
Molecular Weight |
679.7 g/mol |
IUPAC Name |
2-[(2S)-4-[(7R)-7-[3-amino-2-fluoro-5-methyl-6-(trifluoromethyl)phenyl]-2-[[(2R,8S)-2-fluoro-1,2,3,5,6,7-hexahydropyrrolizin-8-yl]methoxy]-7,8-dihydro-5H-pyrano[4,3-d]pyrimidin-4-yl]-1-(2-fluoroprop-2-enoyl)piperazin-2-yl]acetonitrile |
InChI |
InChI=1S/C32H35F6N7O3/c1-17-10-22(40)27(35)25(26(17)32(36,37)38)24-11-23-21(15-47-24)28(43-8-9-45(29(46)18(2)33)20(14-43)4-6-39)42-30(41-23)48-16-31-5-3-7-44(31)13-19(34)12-31/h10,19-20,24H,2-5,7-9,11-16,40H2,1H3/t19-,20+,24-,31+/m1/s1 |
InChI Key |
JOLORSRKBHXPFT-KIQNBNRRSA-N |
Isomeric SMILES |
CC1=CC(=C(C(=C1C(F)(F)F)[C@H]2CC3=C(CO2)C(=NC(=N3)OC[C@@]45CCCN4C[C@@H](C5)F)N6CCN([C@H](C6)CC#N)C(=O)C(=C)F)F)N |
Canonical SMILES |
CC1=CC(=C(C(=C1C(F)(F)F)C2CC3=C(CO2)C(=NC(=N3)OCC45CCCN4CC(C5)F)N6CCN(C(C6)CC#N)C(=O)C(=C)F)F)N |
Origin of Product |
United States |
Disclaimer and Information on In-Vitro Research Products
Please be aware that all articles and product information presented on BenchChem are intended solely for informational purposes. The products available for purchase on BenchChem are specifically designed for in-vitro studies, which are conducted outside of living organisms. In-vitro studies, derived from the Latin term "in glass," involve experiments performed in controlled laboratory settings using cells or tissues. It is important to note that these products are not categorized as medicines or drugs, and they have not received approval from the FDA for the prevention, treatment, or cure of any medical condition, ailment, or disease. We must emphasize that any form of bodily introduction of these products into humans or animals is strictly prohibited by law. It is essential to adhere to these guidelines to ensure compliance with legal and ethical standards in research and experimentation.