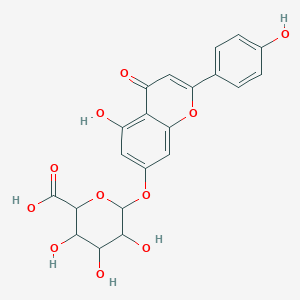
Apigenin 7-glucuronide
Overview
Description
Apigenin 7-glucuronide (C₂₁H₁₈O₁₁, molecular weight 446.36) is a flavonoid-7-O-glucuronide, characterized by a glucuronic acid moiety attached to the 7-hydroxy group of apigenin (4′,5,7-trihydroxyflavone) . It is naturally found in plants such as Phlomis tuberosa, Scutellaria species, and Galeopsis tetrahit . Its extraction and quantification often involve ethanol/water solvents or advanced techniques like ultrasound-assisted extraction .
Preparation Methods
Synthetic Routes and Reaction Conditions
The synthesis of apigenin 7-glucuronide typically involves the glucuronidation of apigenin. This process can be achieved through enzymatic or chemical methods. Enzymatic glucuronidation involves the use of uridine diphosphate-glucuronosyltransferases (UGTs) to transfer glucuronic acid to apigenin . Chemical synthesis, on the other hand, involves the use of glucuronic acid derivatives and catalysts under specific reaction conditions .
Industrial Production Methods
Industrial production of this compound often relies on biotechnological approaches, including the use of genetically engineered microorganisms that express UGTs. These microorganisms can efficiently convert apigenin to this compound under controlled fermentation conditions .
Chemical Reactions Analysis
Types of Reactions
Apigenin 7-glucuronide undergoes various chemical reactions, including:
Oxidation: This reaction can lead to the formation of quinones and other oxidized derivatives.
Reduction: Reduction reactions can convert the compound to its corresponding reduced forms.
Substitution: Nucleophilic substitution reactions can occur at specific positions on the flavonoid ring.
Common Reagents and Conditions
Common reagents used in these reactions include oxidizing agents like hydrogen peroxide, reducing agents like sodium borohydride, and nucleophiles like thiols. The reactions are typically carried out under mild to moderate conditions to preserve the integrity of the flavonoid structure .
Major Products Formed
The major products formed from these reactions include various oxidized, reduced, and substituted derivatives of this compound. These products can exhibit different pharmacological properties compared to the parent compound .
Scientific Research Applications
Anti-Inflammatory Properties
Apigenin 7-glucuronide exhibits notable anti-inflammatory effects, making it a candidate for therapeutic applications in inflammatory diseases. A study demonstrated that this compound inhibited lipopolysaccharide (LPS)-induced inflammatory responses in RAW 264.7 macrophages. It suppressed the production of pro-inflammatory cytokines such as nitric oxide (NO), prostaglandin E2 (PGE2), and tumor necrosis factor-alpha (TNF-α) in a dose-dependent manner without affecting cell viability . Furthermore, it inhibited the expression of inducible nitric oxide synthase (iNOS) and cyclooxygenase-2 (COX-2), which are critical mediators of inflammation .
Case Study: In Vivo Effects
In an animal model, this compound protected mice from LPS-induced endotoxin shock by reducing pro-inflammatory cytokine production . This suggests its potential as a dietary supplement for health promotion and as an anti-inflammatory agent.
Antioxidant Activity
The antioxidant properties of this compound have been explored in various studies, highlighting its ability to scavenge free radicals and reduce oxidative stress. Research indicates that the antioxidant activity of apigenin increases upon hydrolysis from its glucoside form . This raises questions about the relative efficacy of this compound compared to its aglycone form in therapeutic contexts.
Anticancer Effects
This compound has shown promise in cancer research, particularly concerning its cytotoxic effects on cancer cells. It was found to be more effective than apigenin in inducing cell death in colon cancer HCT116 cells, demonstrating significant cytotoxicity at lower concentrations . Additionally, it exhibited antifungal activity against various Candida species, which is relevant for patients with compromised immune systems .
Case Study: Antifungal Activity
A comparative study on the antifungal activity of this compound versus apigenin revealed that this compound had a lower minimum inhibitory concentration (MIC) against Candida strains, indicating stronger antifungal properties . The mechanism involved disruption of membrane integrity more rapidly than apigenin itself.
Metabolism and Bioavailability
Understanding the metabolism of this compound is crucial for evaluating its bioavailability and therapeutic potential. Studies have shown that flavonoids like apigenin undergo extensive glucuronidation via uridine 5′-diphosphate glucuronosyltransferase enzymes in humans, which affects their pharmacokinetics and bioactivity . Specifically, this compound is one of the primary metabolites formed during this process, emphasizing the importance of studying its effects in vivo rather than relying solely on aglycone forms.
Data Table: Summary of Biological Activities
Mechanism of Action
The mechanism of action of apigenin 7-glucuronide involves its interaction with various molecular targets and pathways. It exerts its effects by modulating signaling pathways such as the MAPK and NF-κB pathways, which are involved in inflammation and cell proliferation . The compound also inhibits the activity of enzymes like cyclooxygenase-2 (COX-2) and inducible nitric oxide synthase (iNOS), reducing the production of pro-inflammatory mediators .
Comparison with Similar Compounds
Flavonoid-7-O-glucuronides share structural similarities but differ in aglycone structure, biological activity, and natural sources. Below is a detailed comparison:
Structural and Functional Comparisons
Key Differences in Bioactivity
- Cholesterol-Lowering Effects : Luteolin 7-glucuronide demonstrates significant dose-dependent cholesterol reduction in P. frutescens extracts, though synergies with protocatechuic acid and chlorogenic acid enhance its efficacy . In contrast, this compound’s primary bioactivity revolves around MMP inhibition, critical in cancer metastasis .
- Extraction Efficiency: Luteolin 7-glucuronide yield peaks at 50% ethanol, but higher ethanol concentrations reduce its content while maintaining cholesterol-lowering effects due to co-extracted phenolics . This compound, however, shows increased yield with ultrasound-assisted methods in artichoke by-products .
- Structural Impact on Function : The absence of a 3′-OH group in apigenin (vs. luteolin) reduces antioxidant capacity but enhances specificity for MMP-13 inhibition .
Co-Occurrence in Plant Sources
- Chrysanthemum species contain both this compound and luteolin 7-glucuronide, with the latter being more abundant .
- Scutellaria spp. produce this compound alongside baicalin and wogonin 7-glucuronide, highlighting metabolic pathway similarities .
Research Implications and Gaps
- Synergistic Effects: Luteolin 7-glucuronide’s cholesterol-lowering action is amplified by co-existing phenolics like chlorogenic acid, suggesting multi-component formulations for hyperlipidemia treatment . This compound’s MMP inhibition warrants further in vivo studies for therapeutic validation .
- Analytical Challenges : Distinguishing 7-glucuronides from isomers (e.g., 5-glucuronides) requires advanced chromatography; luteolin 7-glucuronide and this compound share similar UV spectra but differ in retention times .
Q & A
Basic Research Questions
Q. How can researchers confirm the identity of apigenin 7-glucuronide in plant extracts?
- Methodological Answer : Use high-performance liquid chromatography (HPLC) with UV detection at 266–338 nm for preliminary identification, as this compound exhibits distinct UV absorption peaks . Confirm structural identity via nuclear magnetic resonance (NMR) spectroscopy, particularly observing characteristic proton signals (e.g., δ 6.46 ppm for H-6 and δ 7.95 ppm for H-2′/6′) and carbon signals (e.g., δ 71.29 ppm for glucuronide C-4″) . Cross-validate with a primary reference standard (≥98% purity) using retention time and spectral matching .
Q. What protocols are recommended for quantifying this compound in biological fluids?
- Methodological Answer : Employ liquid chromatography-mass spectrometry (LC-MS) with electrospray ionization (ESI) in negative ion mode for high sensitivity. Use deuterated internal standards (e.g., apigenin-d6 glucuronide) to correct for matrix effects. Calibrate with spiked plasma/serum samples (0.1–50 µM range) and validate recovery rates (≥85%) .
Q. What are the primary natural sources of this compound?
- Methodological Answer : Extract from plants such as Lactuca serriola (wild lettuce), Ajuga multiflora, and Verbena officinalis using methanol-water (70:30 v/v) with ultrasonication. Confirm presence via HPLC-UV and compare with authenticated voucher specimens .
Q. How should this compound be stored to ensure stability?
- Methodological Answer : Store lyophilized powder at –20°C in airtight, light-resistant containers. For solutions, use pH 7.4 phosphate-buffered saline (PBS) and avoid repeated freeze-thaw cycles. Monitor degradation via HPLC every 6 months .
Advanced Research Questions
Q. How can researchers resolve discrepancies in reported bioactivity of this compound across studies?
- Methodological Answer : Address variability by standardizing:
- Purity : Use ≥98% purity (HPLC-validated) to exclude confounding impurities .
- Structural analogs : Differentiate from luteolin 7-glucuronide (which shares similar UV profiles but distinct NMR signals) .
- Experimental models : Compare results across cell lines (e.g., HepG2 vs. Caco-2) and animal models (e.g., hyperlipidemic mice vs. zebrafish) .
Q. What experimental designs optimize the study of this compound’s MMP inhibitory activity?
- Methodological Answer : Use recombinant MMP isoforms (e.g., MMP-3, MMP-13) in fluorogenic substrate assays. Pre-incubate this compound (0.1–100 µM) with enzymes for 30 min at 37°C. Calculate IC50 values via nonlinear regression (e.g., MMP-13 IC50 = 0.27 µM) and validate with zymography in collagenase-treated tissues .
Q. How does this compound’s glucuronidation affect its pharmacokinetics?
- Methodological Answer : Administer orally to rodents and collect plasma at timed intervals. Analyze metabolites using LC-MS/MS to distinguish this compound from its aglycone (apigenin). Note that glucuronidation reduces intestinal absorption but enhances renal excretion, requiring dose adjustments in bioavailability studies .
Q. What strategies improve the extraction yield of this compound from complex matrices?
- Methodological Answer : Optimize solvent polarity (e.g., ethyl acetate for partial purification) followed by semi-preparative HPLC (C18 column, 5 µm, 10 × 250 mm) with isocratic elution (20% acetonitrile/0.1% formic acid). Achieve ≥95% recovery by spiking with this compound-d4 as a tracer .
Q. How can molecular docking elucidate this compound’s interaction with therapeutic targets?
- Methodological Answer : Dock the compound into MMP-13’s active site (PDB ID: 3K7Z) using AutoDock Vina. Parameterize glucuronic acid charges with AM1-BCC and validate binding poses via molecular dynamics (100 ns simulations). Compare binding energies with known inhibitors (e.g., ilomastat) to prioritize targets .
Q. What in vivo models are suitable for studying this compound’s cholesterol-lowering effects?
- Methodological Answer : Use high-fat diet (HFD)-fed mice, administering 10–50 mg/kg/day this compound orally for 4 weeks. Measure serum LDL/HDL ratios and hepatic HMG-CoA reductase expression via qPCR. Cross-validate with Perilla frutescens extracts rich in the compound .
Q. Data Contradiction Analysis
Q. Why do some studies report weak antioxidant activity for this compound despite its structural similarity to active flavonoids?
- Analysis : The glucuronic acid moiety reduces membrane permeability, limiting intracellular antioxidant effects. Test in cell-free systems (e.g., DPPH/ABTS assays) vs. cellular models (e.g., H2O2-stressed HepG2) to differentiate direct scavenging from indirect mechanisms (e.g., Nrf2 pathway modulation) .
Q. How can conflicting results on this compound’s stability in gastrointestinal fluids be reconciled?
- Analysis : Simulate gastric (pH 2.0, pepsin) and intestinal (pH 7.4, pancreatin) conditions separately. Use LC-MS to quantify degradation products (e.g., apigenin aglycone). Note that stability varies with bile salt concentrations, requiring physiologically relevant in vitro models (e.g., TIM-1 system) .
Properties
IUPAC Name |
3,4,5-trihydroxy-6-[5-hydroxy-2-(4-hydroxyphenyl)-4-oxochromen-7-yl]oxyoxane-2-carboxylic acid | |
---|---|---|
Details | Computed by LexiChem 2.6.6 (PubChem release 2019.06.18) | |
Source | PubChem | |
URL | https://pubchem.ncbi.nlm.nih.gov | |
Description | Data deposited in or computed by PubChem | |
InChI |
InChI=1S/C21H18O11/c22-9-3-1-8(2-4-9)13-7-12(24)15-11(23)5-10(6-14(15)31-13)30-21-18(27)16(25)17(26)19(32-21)20(28)29/h1-7,16-19,21-23,25-27H,(H,28,29) | |
Details | Computed by InChI 1.0.5 (PubChem release 2019.06.18) | |
Source | PubChem | |
URL | https://pubchem.ncbi.nlm.nih.gov | |
Description | Data deposited in or computed by PubChem | |
InChI Key |
JBFOLLJCGUCDQP-UHFFFAOYSA-N | |
Details | Computed by InChI 1.0.5 (PubChem release 2019.06.18) | |
Source | PubChem | |
URL | https://pubchem.ncbi.nlm.nih.gov | |
Description | Data deposited in or computed by PubChem | |
Canonical SMILES |
C1=CC(=CC=C1C2=CC(=O)C3=C(C=C(C=C3O2)OC4C(C(C(C(O4)C(=O)O)O)O)O)O)O | |
Details | Computed by OEChem 2.1.5 (PubChem release 2019.06.18) | |
Source | PubChem | |
URL | https://pubchem.ncbi.nlm.nih.gov | |
Description | Data deposited in or computed by PubChem | |
Molecular Formula |
C21H18O11 | |
Details | Computed by PubChem 2.1 (PubChem release 2019.06.18) | |
Source | PubChem | |
URL | https://pubchem.ncbi.nlm.nih.gov | |
Description | Data deposited in or computed by PubChem | |
Molecular Weight |
446.4 g/mol | |
Details | Computed by PubChem 2.1 (PubChem release 2021.05.07) | |
Source | PubChem | |
URL | https://pubchem.ncbi.nlm.nih.gov | |
Description | Data deposited in or computed by PubChem | |
Retrosynthesis Analysis
AI-Powered Synthesis Planning: Our tool employs the Template_relevance Pistachio, Template_relevance Bkms_metabolic, Template_relevance Pistachio_ringbreaker, Template_relevance Reaxys, Template_relevance Reaxys_biocatalysis model, leveraging a vast database of chemical reactions to predict feasible synthetic routes.
One-Step Synthesis Focus: Specifically designed for one-step synthesis, it provides concise and direct routes for your target compounds, streamlining the synthesis process.
Accurate Predictions: Utilizing the extensive PISTACHIO, BKMS_METABOLIC, PISTACHIO_RINGBREAKER, REAXYS, REAXYS_BIOCATALYSIS database, our tool offers high-accuracy predictions, reflecting the latest in chemical research and data.
Strategy Settings
Precursor scoring | Relevance Heuristic |
---|---|
Min. plausibility | 0.01 |
Model | Template_relevance |
Template Set | Pistachio/Bkms_metabolic/Pistachio_ringbreaker/Reaxys/Reaxys_biocatalysis |
Top-N result to add to graph | 6 |
Feasible Synthetic Routes
Disclaimer and Information on In-Vitro Research Products
Please be aware that all articles and product information presented on BenchChem are intended solely for informational purposes. The products available for purchase on BenchChem are specifically designed for in-vitro studies, which are conducted outside of living organisms. In-vitro studies, derived from the Latin term "in glass," involve experiments performed in controlled laboratory settings using cells or tissues. It is important to note that these products are not categorized as medicines or drugs, and they have not received approval from the FDA for the prevention, treatment, or cure of any medical condition, ailment, or disease. We must emphasize that any form of bodily introduction of these products into humans or animals is strictly prohibited by law. It is essential to adhere to these guidelines to ensure compliance with legal and ethical standards in research and experimentation.