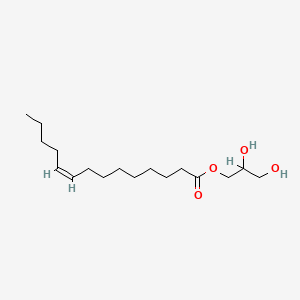
Monomyristolein
Overview
Description
Monomyristolein, also known as 7Z-monomyristolein or 7.7 MAG, is a type of lipid used in lipid cubic phase (LCP) crystallization . Its molecular formula is C17H32O4 . It is often used in structural solutions, including the NapA sodium/proton antiporter .
Molecular Structure Analysis
Monomyristolein has a molecular weight of 300.43 . The temperature-composition phase diagram of Monomyristolein in water was constructed using x-ray diffraction . The phases identified in the system include the lamellar crystalline phase, the lamellar liquid crystalline phase, the fluid isotropic phase, and two inverted cubic phases .Physical And Chemical Properties Analysis
Monomyristolein forms a cubic phase with larger water channels than the more common 9.9 MAG . The cubic-to-lamellar transition rate decreases with increasing sample hydration . Additionally, the transition proceeds more rapidly at an incubation temperature of 25°C compared to that at 0°C .Scientific Research Applications
1. Water Molecule Behavior and Hofmeister Effect
Research has revealed intriguing aspects of water molecule orientation at the surface of the nonionic surfactant monomyristolein. This study by Hishida et al. (2015) utilized heterodyne-detected vibrational sum frequency generation spectroscopy to explore how monomyristolein influences the orientation of water molecules. They discovered that water molecules at the surface orient with their hydrogen atoms pointing to the bulk. Moreover, the extent of this orientation was significantly affected by the presence of monovalent ions, especially anions, providing insights into the mechanism of the Hofmeister effect (Hishida et al., 2015).
2. Transdermal Vaccine Delivery
In the realm of pharmaceuticals, monomyristolein has been explored as a skin penetration enhancer for transdermal drug delivery. A study by Kitaoka et al. (2020) demonstrated the effectiveness of monoolein, a relative of monomyristolein, in enhancing the skin permeation of powder proteins when dissolved in an oil vehicle. This finding is crucial for the development of new methods for transdermal vaccine delivery, offering a non-invasive alternative to traditional injection methods (Kitaoka et al., 2020).
3. Cancer Treatment Applications
Monomyristin has been investigated for its potential in treating cervical cancer. Boondireke et al. (2019) studied the encapsulation of monomyristin into nanoparticles to enhance its efficacy against cervical cancer cells. Their research showed that encapsulating monomyristin increased its cytotoxicity against cancer cells at lower doses, highlighting its potential as a targeted cancer therapy (Boondireke et al., 2019).
4. Antibacterial and Antifungal Properties
Research by Nurmala et al. (2018) into monoacylglycerol derivatives, including monomyristin, revealed their potent antibacterial and antifungal properties. The study found that monomyristin exhibited significant antibacterial activity against various bacterial strains and antifungal activity against Candida albicans. This suggests its potential use in developing new antibacterial and antifungal agents (Nurmala et al., 2018).
properties
IUPAC Name |
2,3-dihydroxypropyl (Z)-tetradec-9-enoate | |
---|---|---|
Source | PubChem | |
URL | https://pubchem.ncbi.nlm.nih.gov | |
Description | Data deposited in or computed by PubChem | |
InChI |
InChI=1S/C17H32O4/c1-2-3-4-5-6-7-8-9-10-11-12-13-17(20)21-15-16(19)14-18/h5-6,16,18-19H,2-4,7-15H2,1H3/b6-5- | |
Source | PubChem | |
URL | https://pubchem.ncbi.nlm.nih.gov | |
Description | Data deposited in or computed by PubChem | |
InChI Key |
ARCRKLOZHGPFFJ-WAYWQWQTSA-N | |
Source | PubChem | |
URL | https://pubchem.ncbi.nlm.nih.gov | |
Description | Data deposited in or computed by PubChem | |
Canonical SMILES |
CCCCC=CCCCCCCCC(=O)OCC(CO)O | |
Source | PubChem | |
URL | https://pubchem.ncbi.nlm.nih.gov | |
Description | Data deposited in or computed by PubChem | |
Isomeric SMILES |
CCCC/C=C\CCCCCCCC(=O)OCC(CO)O | |
Source | PubChem | |
URL | https://pubchem.ncbi.nlm.nih.gov | |
Description | Data deposited in or computed by PubChem | |
Molecular Formula |
C17H32O4 | |
Source | PubChem | |
URL | https://pubchem.ncbi.nlm.nih.gov | |
Description | Data deposited in or computed by PubChem | |
Molecular Weight |
300.4 g/mol | |
Source | PubChem | |
URL | https://pubchem.ncbi.nlm.nih.gov | |
Description | Data deposited in or computed by PubChem | |
CAS RN |
56399-71-4 | |
Record name | Monomyristolein | |
Source | ChemIDplus | |
URL | https://pubchem.ncbi.nlm.nih.gov/substance/?source=chemidplus&sourceid=0056399714 | |
Description | ChemIDplus is a free, web search system that provides access to the structure and nomenclature authority files used for the identification of chemical substances cited in National Library of Medicine (NLM) databases, including the TOXNET system. | |
Retrosynthesis Analysis
AI-Powered Synthesis Planning: Our tool employs the Template_relevance Pistachio, Template_relevance Bkms_metabolic, Template_relevance Pistachio_ringbreaker, Template_relevance Reaxys, Template_relevance Reaxys_biocatalysis model, leveraging a vast database of chemical reactions to predict feasible synthetic routes.
One-Step Synthesis Focus: Specifically designed for one-step synthesis, it provides concise and direct routes for your target compounds, streamlining the synthesis process.
Accurate Predictions: Utilizing the extensive PISTACHIO, BKMS_METABOLIC, PISTACHIO_RINGBREAKER, REAXYS, REAXYS_BIOCATALYSIS database, our tool offers high-accuracy predictions, reflecting the latest in chemical research and data.
Strategy Settings
Precursor scoring | Relevance Heuristic |
---|---|
Min. plausibility | 0.01 |
Model | Template_relevance |
Template Set | Pistachio/Bkms_metabolic/Pistachio_ringbreaker/Reaxys/Reaxys_biocatalysis |
Top-N result to add to graph | 6 |
Feasible Synthetic Routes
Q & A
Q1: What is the impact of salts on the structural organization of monomyristolein in solution?
A: Monomyristolein, a nonionic surfactant, forms lamellar structures in water. Research indicates that the presence of salts can significantly influence the organization of these structures. Specifically, the type of anion present has a more pronounced effect compared to the cation. [] This effect is attributed to the heterogeneous distribution of ions around the monomyristolein molecules, influencing the orientation of water molecules at the interface. []
Q2: How does the molecular structure of water near the surface of monomyristolein change in the presence of salts?
A: Studies utilizing heterodyne-detected vibrational sum frequency generation spectroscopy reveal that water molecules at the monomyristolein surface orient themselves with their hydrogen atoms pointing towards the bulk solution. [] Interestingly, the presence of salts, particularly anions, can enhance this orientation effect. [] This phenomenon is linked to the Hofmeister series and suggests a connection between ion-specific effects and the organization of water molecules at the surfactant interface. []
Q3: What resources are available to researchers interested in studying the phase behavior of monomyristolein?
A: The scientific community has developed detailed temperature-composition phase diagrams for monomyristolein in water. [] This valuable resource helps researchers understand the equilibrium and metastable phases that monomyristolein can adopt under various conditions, facilitating further investigation into its properties and potential applications. []
Disclaimer and Information on In-Vitro Research Products
Please be aware that all articles and product information presented on BenchChem are intended solely for informational purposes. The products available for purchase on BenchChem are specifically designed for in-vitro studies, which are conducted outside of living organisms. In-vitro studies, derived from the Latin term "in glass," involve experiments performed in controlled laboratory settings using cells or tissues. It is important to note that these products are not categorized as medicines or drugs, and they have not received approval from the FDA for the prevention, treatment, or cure of any medical condition, ailment, or disease. We must emphasize that any form of bodily introduction of these products into humans or animals is strictly prohibited by law. It is essential to adhere to these guidelines to ensure compliance with legal and ethical standards in research and experimentation.