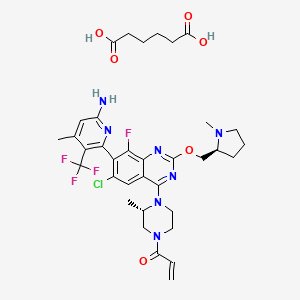
Divarasib adipate
- Click on QUICK INQUIRY to receive a quote from our team of experts.
- With the quality product at a COMPETITIVE price, you can focus more on your research.
Overview
Description
Divarasib adipate is a potent and selective inhibitor of the Kirsten Rat Sarcoma viral oncogene homolog (KRAS) G12C mutation. This mutation is a common oncogenic driver found in various cancers, including non-small cell lung cancer (NSCLC) and colorectal cancer (CRC). This compound has shown promising efficacy in preclinical and clinical studies, making it a potential game-changer in the treatment landscape for KRAS G12C-mutated cancers .
Preparation Methods
Synthetic Routes and Reaction Conditions: Divarasib adipate is synthesized through a series of chemical reactions that involve the formation of covalent bonds with the KRAS G12C protein. The synthetic route typically includes the following steps:
Formation of the Core Structure: The core structure of divarasib is synthesized using a combination of organic reactions, including nucleophilic substitution and cyclization reactions.
Functionalization: The core structure is then functionalized with various chemical groups to enhance its potency and selectivity. This step involves the use of reagents such as halogenating agents and protecting groups.
Adipate Formation: The final step involves the formation of the adipate salt by reacting divarasib with adipic acid under controlled conditions.
Industrial Production Methods: Industrial production of this compound involves scaling up the synthetic route to produce the compound in large quantities. This process requires optimization of reaction conditions, purification methods, and quality control measures to ensure the consistency and purity of the final product .
Chemical Reactions Analysis
Types of Reactions: Divarasib adipate undergoes various chemical reactions, including:
Oxidation: Divarasib can be oxidized under specific conditions to form oxidized derivatives.
Reduction: Reduction reactions can be used to modify the functional groups on divarasib.
Common Reagents and Conditions:
Oxidizing Agents: Hydrogen peroxide, potassium permanganate.
Reducing Agents: Sodium borohydride, lithium aluminum hydride.
Substitution Reagents: Halogenating agents, nucleophiles such as amines and thiols.
Major Products: The major products formed from these reactions include various derivatives of divarasib that retain or enhance its inhibitory activity against KRAS G12C .
Scientific Research Applications
Divarasib adipate has a wide range of scientific research applications, including:
Chemistry: Used as a tool compound to study the structure-activity relationship of KRAS G12C inhibitors.
Biology: Employed in cellular and molecular biology studies to investigate the role of KRAS G12C in cancer progression.
Medicine: Undergoing clinical trials for the treatment of KRAS G12C-mutated cancers, including NSCLC and CRC.
Industry: Potential use in the development of targeted cancer therapies and personalized medicine
Mechanism of Action
Divarasib adipate exerts its effects by covalently binding to the KRAS G12C protein, locking it in an inactive state. This prevents the protein from activating downstream signaling pathways that promote cancer cell proliferation and survival. The molecular targets of this compound include the KRAS G12C protein and associated signaling pathways such as the MAPK and PI3K pathways .
Comparison with Similar Compounds
Sotorasib: Another KRAS G12C inhibitor approved for the treatment of NSCLC.
Adagrasib: A KRAS G12C inhibitor with clinical benefits in NSCLC and CRC
Divarasib adipate’s unique properties and promising clinical results make it a valuable addition to the arsenal of targeted cancer therapies.
Properties
CAS No. |
2762240-36-6 |
---|---|
Molecular Formula |
C35H42ClF4N7O6 |
Molecular Weight |
768.2 g/mol |
IUPAC Name |
1-[(3S)-4-[7-[6-amino-4-methyl-3-(trifluoromethyl)pyridin-2-yl]-6-chloro-8-fluoro-2-[[(2S)-1-methylpyrrolidin-2-yl]methoxy]quinazolin-4-yl]-3-methylpiperazin-1-yl]prop-2-en-1-one;hexanedioic acid |
InChI |
InChI=1S/C29H32ClF4N7O2.C6H10O4/c1-5-21(42)40-9-10-41(16(3)13-40)27-18-12-19(30)22(26-23(29(32,33)34)15(2)11-20(35)36-26)24(31)25(18)37-28(38-27)43-14-17-7-6-8-39(17)4;7-5(8)3-1-2-4-6(9)10/h5,11-12,16-17H,1,6-10,13-14H2,2-4H3,(H2,35,36);1-4H2,(H,7,8)(H,9,10)/t16-,17-;/m0./s1 |
InChI Key |
KUWNSHZGOCXVAI-QJHJCNPRSA-N |
Isomeric SMILES |
C[C@H]1CN(CCN1C2=NC(=NC3=C(C(=C(C=C32)Cl)C4=C(C(=CC(=N4)N)C)C(F)(F)F)F)OC[C@@H]5CCCN5C)C(=O)C=C.C(CCC(=O)O)CC(=O)O |
Canonical SMILES |
CC1CN(CCN1C2=NC(=NC3=C(C(=C(C=C32)Cl)C4=C(C(=CC(=N4)N)C)C(F)(F)F)F)OCC5CCCN5C)C(=O)C=C.C(CCC(=O)O)CC(=O)O |
Origin of Product |
United States |
Disclaimer and Information on In-Vitro Research Products
Please be aware that all articles and product information presented on BenchChem are intended solely for informational purposes. The products available for purchase on BenchChem are specifically designed for in-vitro studies, which are conducted outside of living organisms. In-vitro studies, derived from the Latin term "in glass," involve experiments performed in controlled laboratory settings using cells or tissues. It is important to note that these products are not categorized as medicines or drugs, and they have not received approval from the FDA for the prevention, treatment, or cure of any medical condition, ailment, or disease. We must emphasize that any form of bodily introduction of these products into humans or animals is strictly prohibited by law. It is essential to adhere to these guidelines to ensure compliance with legal and ethical standards in research and experimentation.