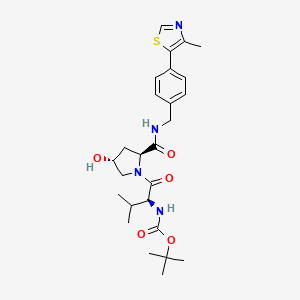
VH032 analogue-2
- Click on QUICK INQUIRY to receive a quote from our team of experts.
- With the quality product at a COMPETITIVE price, you can focus more on your research.
Overview
Description
VH032 analogue-2 is a derivative of VH032, a ligand for the von Hippel-Lindau (VHL) protein. This compound is primarily used in the synthesis of proteolysis-targeting chimeras (PROTACs), which are bifunctional molecules designed to degrade target proteins by recruiting them to the ubiquitin-proteasome system .
Preparation Methods
Synthetic Routes and Reaction Conditions: The synthesis of VH032 analogue-2 involves several key steps:
C–H Arylation of 4-Methylthiazole: This step can be performed using either palladium acetate or Pd-PEPPSI-IPr as catalysts
Protection of Benzylic Amine: N-Boc-L-4-hydroxyproline is used to shield the benzylic amine during the coupling step
Amine Deprotection and Amidation: These steps are crucial for the final formation of this compound
Industrial Production Methods: The industrial production of this compound follows a similar synthetic route but is optimized for large-scale production. This involves the use of high-yielding reaction conditions and efficient purification techniques to ensure the compound’s purity and stability .
Types of Reactions:
Deprotection: this compound undergoes deprotection under acidic conditions to remove protective groups
Amidation: This reaction is used to form the final product from intermediate compounds
Common Reagents and Conditions:
Palladium Acetate or Pd-PEPPSI-IPr: Used as catalysts for C–H arylation
N-Boc-L-4-Hydroxyproline: Used for protecting the benzylic amine
Major Products:
Scientific Research Applications
VH032 analogue-2 has several applications in scientific research:
Chemistry: Used as a ligand for VHL in the synthesis of PROTACs
Biology: Facilitates the degradation of target proteins by recruiting them to the ubiquitin-proteasome system
Medicine: Potential therapeutic applications in cancer treatment by degrading disease-related proteins
Industry: Used in the development of new drugs and therapeutic agents
Mechanism of Action
VH032 analogue-2 acts as a ligand for the VHL protein, recruiting it to form a complex with the target protein. This complex is then ubiquitinated and subsequently degraded by the proteasome . The molecular targets and pathways involved include the ubiquitin-proteasome system and the hypoxia-inducible factor pathway .
Comparison with Similar Compounds
Properties
Molecular Formula |
C26H36N4O5S |
---|---|
Molecular Weight |
516.7 g/mol |
IUPAC Name |
tert-butyl N-[(2S)-1-[(2S,4R)-4-hydroxy-2-[[4-(4-methyl-1,3-thiazol-5-yl)phenyl]methylcarbamoyl]pyrrolidin-1-yl]-3-methyl-1-oxobutan-2-yl]carbamate |
InChI |
InChI=1S/C26H36N4O5S/c1-15(2)21(29-25(34)35-26(4,5)6)24(33)30-13-19(31)11-20(30)23(32)27-12-17-7-9-18(10-8-17)22-16(3)28-14-36-22/h7-10,14-15,19-21,31H,11-13H2,1-6H3,(H,27,32)(H,29,34)/t19-,20+,21+/m1/s1 |
InChI Key |
IBHOCLAWYSUEFY-HKBOAZHASA-N |
Isomeric SMILES |
CC1=C(SC=N1)C2=CC=C(C=C2)CNC(=O)[C@@H]3C[C@H](CN3C(=O)[C@H](C(C)C)NC(=O)OC(C)(C)C)O |
Canonical SMILES |
CC1=C(SC=N1)C2=CC=C(C=C2)CNC(=O)C3CC(CN3C(=O)C(C(C)C)NC(=O)OC(C)(C)C)O |
Origin of Product |
United States |
Disclaimer and Information on In-Vitro Research Products
Please be aware that all articles and product information presented on BenchChem are intended solely for informational purposes. The products available for purchase on BenchChem are specifically designed for in-vitro studies, which are conducted outside of living organisms. In-vitro studies, derived from the Latin term "in glass," involve experiments performed in controlled laboratory settings using cells or tissues. It is important to note that these products are not categorized as medicines or drugs, and they have not received approval from the FDA for the prevention, treatment, or cure of any medical condition, ailment, or disease. We must emphasize that any form of bodily introduction of these products into humans or animals is strictly prohibited by law. It is essential to adhere to these guidelines to ensure compliance with legal and ethical standards in research and experimentation.