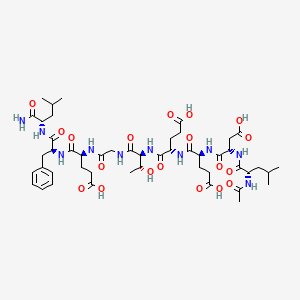
Keap1-Nrf2 probe
- Click on QUICK INQUIRY to receive a quote from our team of experts.
- With the quality product at a COMPETITIVE price, you can focus more on your research.
Overview
Description
The Keap1-Nrf2 probe is a compound used to study the interaction between Kelch-like ECH-associated protein 1 (Keap1) and nuclear factor erythroid 2-related factor 2 (Nrf2). This interaction is crucial in regulating the cellular response to oxidative stress. The Keap1-Nrf2 pathway plays a significant role in protecting cells from damage caused by reactive oxygen species (ROS) and other electrophilic agents .
Preparation Methods
Synthetic Routes and Reaction Conditions
The preparation of Keap1-Nrf2 probes often involves the synthesis of peptides or small molecules that can bind to the Keap1 protein. One common method involves the use of fluorescence polarization (FP) displacement assays and time-resolved fluorescence resonance energy transfer (TR-FRET) to assess the binding affinities of Nrf2 peptide inhibitors . The synthetic routes typically include the following steps:
Peptide Synthesis: The Nrf2 peptide is synthesized using solid-phase peptide synthesis (SPPS) techniques.
Industrial Production Methods
Industrial production methods for Keap1-Nrf2 probes are not well-documented, as these compounds are primarily used in research settings. large-scale peptide synthesis techniques and automated peptide synthesizers can be employed to produce these probes in larger quantities.
Chemical Reactions Analysis
Types of Reactions
The Keap1-Nrf2 probe primarily undergoes non-covalent interactions with the Keap1 protein. These interactions can be studied using various biochemical assays. The probe does not typically undergo traditional chemical reactions such as oxidation, reduction, or substitution.
Common Reagents and Conditions
Common reagents used in the preparation and analysis of Keap1-Nrf2 probes include:
Fluorescent Dyes: FITC is commonly used for labeling the Nrf2 peptide.
Assay Buffers: Buffers are used to maintain the appropriate pH and ionic strength for the binding assays.
Major Products Formed
The major product formed from the interaction of the this compound with the Keap1 protein is a stable complex that can be detected using fluorescence-based assays .
Scientific Research Applications
The Keap1-Nrf2 probe has numerous applications in scientific research, including:
Cancer Research: The Keap1-Nrf2 pathway is implicated in cancer development and progression.
Oxidative Stress Studies: Researchers use the probe to investigate the cellular response to oxidative stress and to identify compounds that can modulate the Keap1-Nrf2 interaction.
Mechanism of Action
The Keap1-Nrf2 probe functions by binding to the Keap1 protein, thereby preventing the degradation of Nrf2. Under normal conditions, Keap1 binds to Nrf2 and targets it for ubiquitination and subsequent proteasomal degradation. When the probe binds to Keap1, it disrupts this interaction, allowing Nrf2 to accumulate in the cytoplasm and translocate to the nucleus. In the nucleus, Nrf2 activates the transcription of antioxidant-responsive genes, which help protect cells from oxidative damage .
Comparison with Similar Compounds
Similar Compounds
Several other compounds are used to study the Keap1-Nrf2 interaction, including:
Bardoxolone methyl: A synthetic triterpenoid that activates Nrf2 by modifying cysteine residues on Keap1.
Sulforaphane: A naturally occurring compound found in cruciferous vegetables that activates Nrf2 by modifying Keap1.
Dimethyl fumarate: An electrophilic compound that activates Nrf2 by modifying Keap1.
Uniqueness
The Keap1-Nrf2 probe is unique in its ability to provide a direct and specific measurement of the Keap1-Nrf2 interaction. Unlike other compounds that activate Nrf2 through indirect mechanisms, the probe allows researchers to study the binding affinity and kinetics of the Keap1-Nrf2 interaction in real-time using fluorescence-based assays .
Properties
Molecular Formula |
C48H72N10O19 |
---|---|
Molecular Weight |
1093.1 g/mol |
IUPAC Name |
(4S)-4-[[2-[[(2S,3R)-2-[[(2S)-2-[[(2S)-2-[[(2S)-2-[[(2S)-2-acetamido-4-methylpentanoyl]amino]-3-carboxypropanoyl]amino]-4-carboxybutanoyl]amino]-4-carboxybutanoyl]amino]-3-hydroxybutanoyl]amino]acetyl]amino]-5-[[(2S)-1-[[(2S)-1-amino-4-methyl-1-oxopentan-2-yl]amino]-1-oxo-3-phenylpropan-2-yl]amino]-5-oxopentanoic acid |
InChI |
InChI=1S/C48H72N10O19/c1-23(2)18-31(41(49)70)55-46(75)33(20-27-10-8-7-9-11-27)56-42(71)28(12-15-36(62)63)52-35(61)22-50-48(77)40(25(5)59)58-44(73)30(14-17-38(66)67)53-43(72)29(13-16-37(64)65)54-47(76)34(21-39(68)69)57-45(74)32(19-24(3)4)51-26(6)60/h7-11,23-25,28-34,40,59H,12-22H2,1-6H3,(H2,49,70)(H,50,77)(H,51,60)(H,52,61)(H,53,72)(H,54,76)(H,55,75)(H,56,71)(H,57,74)(H,58,73)(H,62,63)(H,64,65)(H,66,67)(H,68,69)/t25-,28+,29+,30+,31+,32+,33+,34+,40+/m1/s1 |
InChI Key |
UULDEJYFSBSBCA-PRDVVYQQSA-N |
Isomeric SMILES |
C[C@H]([C@@H](C(=O)NCC(=O)N[C@@H](CCC(=O)O)C(=O)N[C@@H](CC1=CC=CC=C1)C(=O)N[C@@H](CC(C)C)C(=O)N)NC(=O)[C@H](CCC(=O)O)NC(=O)[C@H](CCC(=O)O)NC(=O)[C@H](CC(=O)O)NC(=O)[C@H](CC(C)C)NC(=O)C)O |
Canonical SMILES |
CC(C)CC(C(=O)N)NC(=O)C(CC1=CC=CC=C1)NC(=O)C(CCC(=O)O)NC(=O)CNC(=O)C(C(C)O)NC(=O)C(CCC(=O)O)NC(=O)C(CCC(=O)O)NC(=O)C(CC(=O)O)NC(=O)C(CC(C)C)NC(=O)C |
Origin of Product |
United States |
Disclaimer and Information on In-Vitro Research Products
Please be aware that all articles and product information presented on BenchChem are intended solely for informational purposes. The products available for purchase on BenchChem are specifically designed for in-vitro studies, which are conducted outside of living organisms. In-vitro studies, derived from the Latin term "in glass," involve experiments performed in controlled laboratory settings using cells or tissues. It is important to note that these products are not categorized as medicines or drugs, and they have not received approval from the FDA for the prevention, treatment, or cure of any medical condition, ailment, or disease. We must emphasize that any form of bodily introduction of these products into humans or animals is strictly prohibited by law. It is essential to adhere to these guidelines to ensure compliance with legal and ethical standards in research and experimentation.