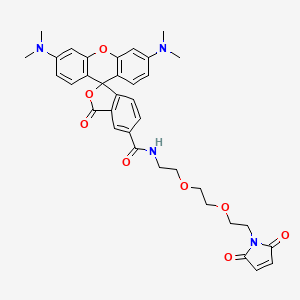
TAMRA-PEG2-Maleimide
- Click on QUICK INQUIRY to receive a quote from our team of experts.
- With the quality product at a COMPETITIVE price, you can focus more on your research.
Overview
Description
TAMRA-PEG2-Maleimide is a fluorescent labeling reagent combining the tetramethylrhodamine (TAMRA) dye with a maleimide-reactive group via a polyethylene glycol (PEG) spacer. Its structure includes:
- TAMRA fluorophore: Excitation/emission maxima at 553/575 nm, enabling red fluorescence detection .
- PEG2 linker: A short PEG chain (2 ethylene glycol units) enhancing solubility and reducing steric hindrance during conjugation .
- Maleimide group: Reacts selectively with thiol (-SH) groups on cysteine residues or other sulfhydryl-containing biomolecules (e.g., peptides, proteins) to form stable thioether bonds .
This compound is widely used in bioconjugation, fluorescence microscopy, and biosensing due to its balanced hydrophilicity, efficient reactivity, and photostability. It is available in reagent-grade purity (≥90%) and is commonly employed in research settings .
Preparation Methods
Synthetic Routes and Reaction Conditions
The synthesis of TAMRA-PEG2-Maleimide involves the conjugation of TAMRA with PEG units and the incorporation of a maleimide group. The process typically starts with the activation of TAMRA, followed by the attachment of PEG units through a series of chemical reactions.
Industrial Production Methods
Industrial production of this compound follows similar synthetic routes but on a larger scale. The process involves the use of automated reactors and purification systems to ensure high yield and purity. The compound is typically produced in bulk and stored under controlled conditions to maintain its stability and reactivity .
Chemical Reactions Analysis
Reaction Mechanism and Chemoselectivity
TAMRA-PEG2-Maleimide undergoes a thiol-Michael addition reaction , forming stable thioether bonds with free sulfhydryl (-SH) groups. The reaction mechanism involves nucleophilic attack by the thiolate ion on the electron-deficient maleimide double bond (Figure 1) . Key features include:
-
pH Sensitivity : Optimal reactivity occurs between pH 6.5–7.5, where thiols exist as thiolate ions (Figure 2) .
-
Chemoselectivity : At pH 7.0, maleimide reacts with thiols ~1,000x faster than with primary amines . Above pH 7.5, amine competition increases due to maleimide hydrolysis .
Table 1: Reaction Rate Comparison at pH 7.0
Substrate | Relative Reaction Rate |
---|---|
Thiols (-SH) | 1,000 |
Amines (-NH₂) | 1 |
Data sourced from |
Competing Reactions and Side Products
While highly specific under optimal conditions, this compound can engage in undesired reactions:
Hydrolysis
Maleimide groups hydrolyze to non-reactive maleamic acid in aqueous solutions, especially at alkaline pH (>8.5) . This limits storage stability and requires fresh preparation for experiments.
Amine Cross-Reactivity
At pH >7.5, primary amines (e.g., lysine residues) compete with thiols, leading to non-specific labeling .
Plasma Protein Interactions
In biological systems, maleimide reacts with plasma thiols (e.g., cysteine, albumin), causing off-target binding and complement activation .
Buffer Conditions
-
Solvents : Reactions proceed efficiently in polar solvents (e.g., water, DMSO) .
-
Reducing Agents : Avoid thiol-containing agents (e.g., DTT, β-mercaptoethanol). TCEP is preferred for disulfide reduction, as it lacks free thiols .
Quenching
Excess maleimide can be neutralized post-conjugation using stoichiometric cysteine :
Table 2: Cysteine Quenching Efficiency
Cysteine:Maleimide Ratio | Albumin Binding Reduction | Complement Activation Reduction |
---|---|---|
1:1 | ~90% | ~95% |
0.5:1 | ~50% | ~60% |
Data adapted from |
Limitations and Mitigation
-
In Vivo Instability : Plasma thiols reduce labeling efficiency. Pre-quenching with cysteine minimizes off-target effects .
-
Hydrolysis : Use fresh reagent and avoid prolonged storage in aqueous buffers .
This compound remains a cornerstone of bioconjugation due to its specificity and versatility. Proper pH control, quenching protocols, and solvent selection are critical for maximizing reaction efficiency and reducing side reactions.
Scientific Research Applications
Key Features and Properties
- Fluorescent Properties : TAMRA dye exhibits bright red-orange fluorescence with an excitation peak around 555 nm and an emission peak at approximately 580 nm. This makes it suitable for sensitive detection in various assays.
- Reactivity : The maleimide group allows for selective conjugation with thiol-containing biomolecules, facilitating targeted labeling and tracking of proteins, peptides, and nucleic acids.
- PEG Spacer : The inclusion of a PEG2 spacer improves solubility in aqueous environments and reduces steric hindrance during conjugation reactions.
Bioconjugation
TAMRA-PEG2-Maleimide is widely used for labeling proteins and peptides. Its ability to react specifically with thiol groups allows researchers to create stable conjugates for various applications:
- Protein Labeling : It can label antibodies or enzymes for detection in assays such as ELISA or Western blotting.
- Peptide Conjugation : The compound is used to attach fluorescent tags to peptides for studying their interactions and functions in biological systems.
Fluorescence Imaging
The bright fluorescence of TAMRA makes it ideal for imaging applications:
- Live Cell Imaging : Researchers utilize this compound to visualize cellular processes in real-time, providing insights into cellular dynamics and interactions.
- Fluorescence Microscopy : It is employed in microscopy techniques to study the localization and distribution of biomolecules within cells or tissues.
Therapeutic Development
In drug development, this compound plays a crucial role:
- Antibody-Drug Conjugates (ADCs) : The compound can be integrated into ADCs, allowing for targeted delivery of cytotoxic drugs to cancer cells while minimizing effects on healthy tissues.
- Diagnostics : It is used in the development of diagnostic agents that can be tracked using fluorescence-based imaging techniques.
Case Study 1: Protein Labeling for Detection
A study demonstrated the effectiveness of this compound in labeling a monoclonal antibody for enhanced detection in flow cytometry. The labeled antibody showed significantly improved sensitivity compared to unlabeled controls, allowing for better discrimination between cell populations.
Case Study 2: Live Cell Imaging
In another research project, this compound was utilized to tag a peptide involved in cell signaling pathways. Fluorescence microscopy revealed dynamic changes in peptide localization during cellular responses to stimuli, providing valuable insights into signaling mechanisms.
Case Study 3: Development of ADCs
Recent advancements highlighted the use of this compound in creating ADCs targeting specific cancer markers. The resulting conjugates exhibited higher efficacy in killing cancer cells while sparing normal cells, showcasing the potential for more effective cancer therapies.
Comparative Data Table
Application Area | Description | Key Benefits |
---|---|---|
Bioconjugation | Labels proteins/peptides for assays | High specificity and stability |
Fluorescence Imaging | Visualizes biomolecules in live cells | Real-time tracking with minimal disturbance |
Therapeutic Development | Integrates into ADCs for targeted drug delivery | Enhanced efficacy with reduced side effects |
Mechanism of Action
The mechanism of action of TAMRA-PEG2-Maleimide involves the formation of covalent bonds with thiol groups on biomolecules. The maleimide group reacts specifically with free thiol groups, forming stable thioether bonds. This reaction is highly efficient and occurs under mild conditions, preserving the integrity of the labeled biomolecules .
Comparison with Similar Compounds
The following table and analysis highlight key distinctions between TAMRA-PEG2-Maleimide and structurally or functionally analogous compounds:
Key Comparative Insights:
PEG Spacer Length :
- This compound’s PEG2 linker offers moderate steric flexibility compared to PEG-free TAMRA-Maleimide, which may hinder conjugation to buried thiols . Conversely, DSPE-PEG-Maleimide (longer PEG chains) enhances solubility and biocompatibility for drug delivery but increases molecular weight .
Reactivity and Stability :
- Maleimide-thiol conjugates in this compound are stable under neutral pH but may hydrolyze in blood plasma (pH 7.4), a limitation shared with DSPE-PEG-Maleimide . In contrast, Methyltetrazine-PEG2-Maleimide’s tetrazine group enables additional bioorthogonal reactions (e.g., with trans-cyclooctenes), broadening application scope .
Fluorescence Properties :
- TAMRA derivatives exhibit superior photostability compared to fluorescein-based probes. However, this compound’s fluorescence intensity is slightly reduced (~10%) compared to PEG-free TAMRA-Maleimide due to PEG’s quenching effects .
Functional Versatility :
- Homo-bifunctional Maleimide-PEG-Maleimide is ideal for creating stable crosslinks but lacks fluorescent tracking capabilities, unlike this compound .
Research Findings and Practical Considerations
- Conjugation Efficiency : this compound achieves >80% labeling efficiency for cysteine-containing proteins under optimized conditions (pH 6.5–7.5, 2-hour reaction) .
- In Vivo Limitations: PEG immunogenicity and maleimide instability in vivo make this compound less suitable for therapeutic applications compared to alternatives like site-specific click chemistry probes .
- Cost and Availability : this compound is commercially available at lower costs (~$200/mg) than specialized reagents like Methyltetrazine-PEG2-Maleimide (\sim$500/mg) .
Biological Activity
TAMRA-PEG2-Maleimide is a fluorescent dye derivative of TAMRA (Tetramethylrhodamine) that incorporates two polyethylene glycol (PEG) units and features a maleimide functional group. This compound is primarily utilized in bioconjugation applications, enabling the labeling of biomolecules such as proteins and peptides for various biological studies. Its unique structure allows for specific interactions with thiol groups, making it an essential tool in molecular biology and biochemistry.
The biological activity of this compound is largely attributed to its ability to form covalent bonds with sulfhydryl groups present in cysteine residues of proteins. This reaction occurs through a Michael addition mechanism, where the maleimide group reacts with the thiol group, leading to stable conjugates. This property is critical for applications in fluorescence microscopy, flow cytometry, and other analytical techniques where tracking protein interactions and dynamics is necessary.
Applications in Research
- Protein Labeling : this compound is extensively used for labeling proteins in live-cell imaging studies. The fluorescent properties enable visualization of protein localization and dynamics within cellular environments.
- Bioconjugation : The compound's maleimide functionality allows for selective conjugation to proteins, which can be exploited in developing targeted therapeutics and diagnostics.
- Fluorescence Studies : The dye's fluorescence characteristics make it suitable for various assays, including fluorescence resonance energy transfer (FRET) experiments, where it can serve as a donor or acceptor.
Study 1: Protein Dynamics
A study investigated the dynamics of a specific protein tagged with this compound in live cells. Researchers observed the localization changes of the protein during cellular stress responses. The results indicated that the tagged protein translocated from the cytoplasm to the nucleus under stress conditions, demonstrating the utility of this compound in studying real-time cellular processes.
Study 2: Targeted Drug Delivery
In another research effort, this compound was employed to create targeted drug delivery systems by conjugating it to therapeutic agents. The study showed that the conjugated drugs exhibited enhanced cellular uptake and specificity towards cancer cells compared to free drugs. This highlights the potential of this compound in developing more effective therapeutic strategies.
Comparative Data Table
Property | This compound | Alternative Dyes |
---|---|---|
Molecular Weight | ~ 600 Da | Varies (e.g., 500-800 Da) |
Fluorescence Emission Peak | 580 nm | Varies (e.g., 550-650 nm) |
Reactivity with Thiols | High | Moderate to High |
Stability in Biological Systems | Moderate | Varies |
Key Findings from Literature
- Fluorescence Characteristics : The compound exhibits strong fluorescence under UV light, making it suitable for sensitive detection methods .
- Cellular Uptake : Studies have shown that proteins labeled with this compound are efficiently internalized by cells, facilitating studies on intracellular trafficking .
- Selectivity and Specificity : The maleimide group provides high selectivity for thiol-containing molecules, minimizing off-target effects during conjugation .
Q & A
Basic Question: How can researchers confirm the successful conjugation of TAMRA-PEG2-Maleimide with thiol-containing biomolecules?
Methodological Answer:
Successful conjugation can be validated using UV-Vis spectroscopy to detect TAMRA's characteristic absorbance peaks (e.g., ~550 nm). A spectral shift or quenching indicates covalent bonding. Additionally, SDS-PAGE can reveal increased molecular weight of the conjugate compared to the unmodified biomolecule. For quantitative analysis, HPLC with fluorescence detection is recommended to separate and quantify conjugated vs. unreacted species .
Advanced Question: What experimental strategies optimize reaction conditions for this compound conjugation to minimize non-specific binding?
Methodological Answer:
Optimize by systematically varying:
- pH (6.5–7.5 to balance maleimide reactivity and biomolecule stability).
- Temperature (4°C reduces hydrolysis but slows reaction kinetics).
- Molar ratios (e.g., 1:1 to 1:5 maleimide-to-thiol ratios).
Monitor efficiency via HPLC or fluorescence polarization assays . Include negative controls (e.g., reactions without thiols) to assess non-specific interactions. Pre-treat biomolecules with tris(2-carboxyethyl)phosphine (TCEP) to reduce disulfide bonds and enhance thiol accessibility .
Basic Question: What analytical techniques are used to determine the molecular weight and purity of this compound?
Methodological Answer:
- MALDI-TOF mass spectrometry provides exact molecular weight verification, critical for confirming the PEG spacer length (4 units as per structural data).
- Reverse-phase HPLC with a C18 column assesses purity by separating unreacted starting materials or degradation products.
- Nuclear Magnetic Resonance (NMR) can further validate structural integrity, focusing on maleimide proton signals (~6.7 ppm) .
Advanced Question: How should researchers address discrepancies in reported conjugation efficiencies of this compound across studies?
Methodological Answer:
Discrepancies often arise from:
- Buffer composition (e.g., presence of competing thiols in Tris buffers).
- Purification methods (e.g., dialysis vs. size-exclusion chromatography).
To resolve contradictions:
Replicate experiments under controlled conditions (standardized pH, temperature, and reactant ratios).
Use thiol quantification assays (e.g., Ellman’s reagent) to verify active thiol availability.
Compare results against a reference study with openly shared protocols to isolate variables .
Basic Question: What role does the PEG spacer play in this compound’s functionality?
Methodological Answer:
The PEG spacer reduces steric hindrance between TAMRA and the conjugated biomolecule, enhancing labeling efficiency. It also improves solubility in aqueous buffers. To evaluate PEG’s impact:
- Compare conjugation yields using this compound vs. analogs with shorter/longer PEG chains.
- Assess fluorescence quenching in PEG-free controls via spectrofluorometry .
Advanced Question: How can the stability of this compound conjugates be evaluated under physiological conditions?
Methodological Answer:
Perform stability assays by incubating conjugates in phosphate-buffered saline (PBS, pH 7.4) at 37°C. Monitor over time using:
- Gel electrophoresis to detect degradation products.
- Fluorescence resonance energy transfer (FRET) to track bond integrity.
- LC-MS to identify hydrolysis byproducts (e.g., maleamic acid). Include reducing agents (e.g., glutathione) to simulate intracellular environments .
Basic Question: What experimental controls are essential when using this compound in fluorescence-based assays?
Methodological Answer:
Critical controls include:
- Unconjugated this compound to assess autofluorescence.
- Thiol-free reactions to detect non-specific binding.
- Competitive inhibition (e.g., excess free thiols to block conjugation).
Use fluorescence microscopy or flow cytometry to quantify background signals and normalize data .
Advanced Question: What strategies mitigate maleimide-thiol bond instability in long-term this compound applications?
Methodological Answer:
To enhance stability:
- Use TCEP to maintain thiols in reduced states during conjugation.
- Replace maleimide with stable alternatives (e.g., dibromomaleimide or arylthiol-reactive groups).
- Conduct accelerated aging studies (e.g., elevated temperature) to predict shelf-life and optimize storage conditions (e.g., lyophilization in inert buffers) .
Properties
Molecular Formula |
C35H36N4O8 |
---|---|
Molecular Weight |
640.7 g/mol |
IUPAC Name |
3',6'-bis(dimethylamino)-N-[2-[2-[2-(2,5-dioxopyrrol-1-yl)ethoxy]ethoxy]ethyl]-3-oxospiro[2-benzofuran-1,9'-xanthene]-5-carboxamide |
InChI |
InChI=1S/C35H36N4O8/c1-37(2)23-6-9-27-29(20-23)46-30-21-24(38(3)4)7-10-28(30)35(27)26-8-5-22(19-25(26)34(43)47-35)33(42)36-13-15-44-17-18-45-16-14-39-31(40)11-12-32(39)41/h5-12,19-21H,13-18H2,1-4H3,(H,36,42) |
InChI Key |
CIERBKQMSJKNLG-UHFFFAOYSA-N |
Canonical SMILES |
CN(C)C1=CC2=C(C=C1)C3(C4=C(C=C(C=C4)C(=O)NCCOCCOCCN5C(=O)C=CC5=O)C(=O)O3)C6=C(O2)C=C(C=C6)N(C)C |
Origin of Product |
United States |
Disclaimer and Information on In-Vitro Research Products
Please be aware that all articles and product information presented on BenchChem are intended solely for informational purposes. The products available for purchase on BenchChem are specifically designed for in-vitro studies, which are conducted outside of living organisms. In-vitro studies, derived from the Latin term "in glass," involve experiments performed in controlled laboratory settings using cells or tissues. It is important to note that these products are not categorized as medicines or drugs, and they have not received approval from the FDA for the prevention, treatment, or cure of any medical condition, ailment, or disease. We must emphasize that any form of bodily introduction of these products into humans or animals is strictly prohibited by law. It is essential to adhere to these guidelines to ensure compliance with legal and ethical standards in research and experimentation.