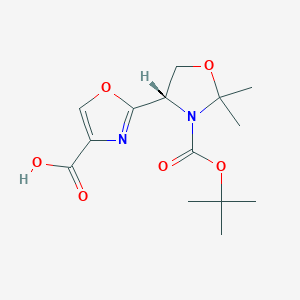
L2H2-6OTD intermediate-2
- Click on QUICK INQUIRY to receive a quote from our team of experts.
- With the quality product at a COMPETITIVE price, you can focus more on your research.
Overview
Description
L2H2-6OTD intermediate-2, also known by its chemical name (S)-2-(3-(tert-butoxycarbonyl)-2,2-dimethyloxazolidin-4-yl)oxazole-4-carboxylic acid, is a synthetic intermediate used in the preparation of telomerase inhibitors . This compound is significant in the field of medicinal chemistry due to its role in the synthesis of anti-cancer agents, particularly those targeting telomerase, an enzyme that plays a crucial role in cellular aging and cancer proliferation .
Preparation Methods
Synthetic Routes and Reaction Conditions
The synthesis of L2H2-6OTD intermediate-2 involves multiple steps, starting from readily available starting materials. The key steps include the formation of the oxazolidinone ring and the subsequent introduction of the oxazole moiety. The reaction conditions typically involve the use of protecting groups, such as tert-butoxycarbonyl, to ensure selective reactions at specific sites .
Industrial Production Methods
Industrial production of this compound follows similar synthetic routes but is optimized for large-scale production. This involves the use of continuous flow reactors and automated systems to ensure high yield and purity. The compound is typically stored under nitrogen at 4°C to prevent degradation .
Chemical Reactions Analysis
Types of Reactions
L2H2-6OTD intermediate-2 undergoes various chemical reactions, including:
Oxidation: The compound can be oxidized to introduce additional functional groups.
Reduction: Reduction reactions can be used to modify the oxazole ring.
Substitution: The oxazole and oxazolidinone rings can undergo substitution reactions to introduce different substituents.
Common Reagents and Conditions
Common reagents used in these reactions include oxidizing agents like potassium permanganate, reducing agents such as lithium aluminum hydride, and various nucleophiles for substitution reactions. The reactions are typically carried out under controlled temperatures and inert atmospheres to prevent unwanted side reactions .
Major Products
The major products formed from these reactions include modified oxazole and oxazolidinone derivatives, which can be further used in the synthesis of more complex molecules, including telomerase inhibitors .
Scientific Research Applications
L2H2-6OTD intermediate-2 is primarily used in the synthesis of telomerase inhibitors, which have significant applications in cancer research. Telomerase inhibitors are being investigated for their potential to limit the proliferation of cancer cells by targeting the enzyme telomerase, which is overexpressed in many types of cancer . Additionally, this compound is used in the development of antibody-drug conjugates (ADCs), which are targeted cancer therapies that deliver cytotoxic agents directly to cancer cells .
Mechanism of Action
The mechanism of action of L2H2-6OTD intermediate-2 involves its role as a precursor in the synthesis of telomerase inhibitors. These inhibitors bind to the telomeric G-quadruplex structures, stabilizing them and preventing the action of telomerase. This leads to the shortening of telomeres and eventual cell death in cancer cells . The molecular targets include the telomeric DNA and the telomerase enzyme, with pathways involving the stabilization of G-quadruplex structures .
Comparison with Similar Compounds
L2H2-6OTD intermediate-2 is unique due to its specific structure, which allows for the selective synthesis of telomerase inhibitors. Similar compounds include other oxazole and oxazolidinone derivatives, such as:
Telomestatin: A natural product that also targets telomeric G-quadruplexes.
TMPyP4: A synthetic porphyrin that stabilizes G-quadruplex structures.
BRACO-19: A synthetic compound that binds to G-quadruplexes and inhibits telomerase.
These compounds share similar mechanisms of action but differ in their chemical structures and specific interactions with telomeric DNA .
Properties
Molecular Formula |
C14H20N2O6 |
---|---|
Molecular Weight |
312.32 g/mol |
IUPAC Name |
2-[(4S)-2,2-dimethyl-3-[(2-methylpropan-2-yl)oxycarbonyl]-1,3-oxazolidin-4-yl]-1,3-oxazole-4-carboxylic acid |
InChI |
InChI=1S/C14H20N2O6/c1-13(2,3)22-12(19)16-9(7-21-14(16,4)5)10-15-8(6-20-10)11(17)18/h6,9H,7H2,1-5H3,(H,17,18)/t9-/m0/s1 |
InChI Key |
ROGULVVSLYGJPQ-VIFPVBQESA-N |
Isomeric SMILES |
CC1(N([C@@H](CO1)C2=NC(=CO2)C(=O)O)C(=O)OC(C)(C)C)C |
Canonical SMILES |
CC1(N(C(CO1)C2=NC(=CO2)C(=O)O)C(=O)OC(C)(C)C)C |
Origin of Product |
United States |
Disclaimer and Information on In-Vitro Research Products
Please be aware that all articles and product information presented on BenchChem are intended solely for informational purposes. The products available for purchase on BenchChem are specifically designed for in-vitro studies, which are conducted outside of living organisms. In-vitro studies, derived from the Latin term "in glass," involve experiments performed in controlled laboratory settings using cells or tissues. It is important to note that these products are not categorized as medicines or drugs, and they have not received approval from the FDA for the prevention, treatment, or cure of any medical condition, ailment, or disease. We must emphasize that any form of bodily introduction of these products into humans or animals is strictly prohibited by law. It is essential to adhere to these guidelines to ensure compliance with legal and ethical standards in research and experimentation.