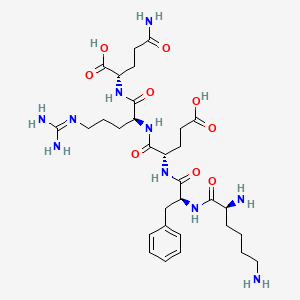
Lys-Phe-Glu-Arg-Gln
- Click on QUICK INQUIRY to receive a quote from our team of experts.
- With the quality product at a COMPETITIVE price, you can focus more on your research.
Overview
Description
Lys-Phe-Glu-Arg-Gln is a pentapeptide sequence that plays a significant role in chaperone-mediated autophagy (CMA). This process involves the selective degradation of cytosolic proteins in lysosomes, which is crucial for maintaining cellular homeostasis and preventing the accumulation of damaged proteins . The sequence this compound is recognized by heat shock cognate protein 70 (HSC70), which facilitates the transport of these proteins into lysosomes for degradation .
Preparation Methods
Synthetic Routes and Reaction Conditions
The synthesis of Lys-Phe-Glu-Arg-Gln can be achieved through solid-phase peptide synthesis (SPPS). This method involves the sequential addition of protected amino acids to a growing peptide chain anchored to a solid resin. The process typically includes the following steps:
Attachment of the first amino acid: to the resin.
Deprotection: of the amino acid’s protecting group.
Coupling: of the next amino acid using coupling reagents like HBTU or DIC.
Repetition: of deprotection and coupling steps until the desired peptide sequence is obtained.
Cleavage: of the peptide from the resin and removal of side-chain protecting groups using a cleavage cocktail (e.g., TFA, water, and scavengers).
Industrial Production Methods
Industrial production of this compound follows similar principles as laboratory synthesis but on a larger scale. Automated peptide synthesizers are often used to increase efficiency and consistency. The process involves optimizing reaction conditions, such as temperature, solvent, and reagent concentrations, to ensure high yield and purity.
Chemical Reactions Analysis
Types of Reactions
Lys-Phe-Glu-Arg-Gln primarily undergoes hydrolysis and enzymatic degradation. The peptide can be cleaved by proteases, which break the peptide bonds between amino acids. Additionally, it can participate in oxidation-reduction reactions, particularly involving the amino acid residues.
Common Reagents and Conditions
Proteases: Enzymes like trypsin and chymotrypsin can cleave the peptide at specific sites.
Oxidizing agents: Hydrogen peroxide or other mild oxidizing agents can oxidize certain amino acid residues.
Reducing agents: Dithiothreitol (DTT) or β-mercaptoethanol can reduce disulfide bonds if present.
Major Products Formed
The major products formed from the degradation of this compound are smaller peptide fragments and free amino acids. These products can be further metabolized or utilized in various cellular processes.
Scientific Research Applications
Lys-Phe-Glu-Arg-Gln has several applications in scientific research, particularly in the fields of biology and medicine:
Autophagy Research: It is used to study the mechanisms of chaperone-mediated autophagy and its role in cellular homeostasis.
Neurodegenerative Diseases: Research has shown that CMA plays a role in the degradation of proteins associated with neurodegenerative diseases like Parkinson’s disease.
Cancer Research: CMA is involved in the regulation of protein degradation in cancer cells, making it a potential target for cancer therapy.
Drug Development: The peptide sequence is used in the development of drugs that can modulate CMA activity for therapeutic purposes.
Mechanism of Action
The mechanism of action of Lys-Phe-Glu-Arg-Gln involves its recognition by HSC70, a chaperone protein. HSC70 binds to the peptide sequence and facilitates its transport to the lysosomal membrane. The peptide is then translocated into the lysosome, where it undergoes degradation . This process is crucial for the selective degradation of damaged or misfolded proteins, maintaining cellular homeostasis .
Comparison with Similar Compounds
Similar Compounds
Lys-Phe-Glu-Arg-Gln (KFERQ): This sequence is similar to this compound and is also recognized by HSC70 for CMA.
Other KFERQ-like motifs: Peptides containing sequences similar to this compound are also targeted for degradation by CMA.
Uniqueness
This compound is unique due to its specific recognition by HSC70 and its role in chaperone-mediated autophagy. This selective degradation pathway is distinct from other forms of autophagy, such as macroautophagy and microautophagy, making it a valuable target for research and therapeutic applications .
Biological Activity
Lys-Phe-Glu-Arg-Gln (KFERQ) is a pentapeptide that plays a significant role in various biological processes, particularly in protein degradation pathways. This article explores the biological activity of KFERQ, focusing on its mechanisms in cellular proteostasis, its implications in disease, and relevant research findings.
1.1 Chaperone-Mediated Autophagy (CMA)
KFERQ is recognized as a key motif for chaperone-mediated autophagy, a selective degradation pathway for misfolded proteins. Proteins containing the KFERQ sequence are targeted by the chaperone Hsc70, which facilitates their translocation into the lysosome for degradation. This process is crucial for maintaining cellular homeostasis, especially under stress conditions such as nutrient deprivation.
- Key Steps in CMA :
- Binding : Hsc70 binds to the KFERQ motif exposed in misfolded proteins.
- Translocation : The complex interacts with lysosomal membrane protein LAMP-2A, forming a multimeric complex necessary for cargo translocation.
- Degradation : Once inside the lysosome, the protein is degraded by lysosomal enzymes.
1.2 Proteolytic Pathways
KFERQ motifs are also involved in other proteolytic pathways, including ubiquitin-proteasome systems and lysosomal degradation mechanisms. The presence of KFERQ-like sequences can signal proteins for rapid degradation under conditions of cellular stress or damage.
2.1 Role in Disease
The dysregulation of KFERQ-mediated degradation pathways has been implicated in several diseases, particularly neurodegenerative disorders such as Parkinson's disease and Huntington's disease. In these conditions, the accumulation of misfolded proteins can overwhelm the cell's ability to degrade them, leading to cellular toxicity.
- Case Study: Parkinson's Disease
2.2 Physiological Implications
In physiological contexts, KFERQ plays a vital role in regulating protein turnover and cellular responses to stress. For instance, during fasting or nutrient deprivation, increased proteolysis via CMA helps cells adapt by recycling amino acids from damaged proteins .
3. Research Findings
Several studies have investigated the biological activity of KFERQ and its implications:
4. Conclusion
This compound (KFERQ) is a critical motif involved in cellular proteostasis through its role in chaperone-mediated autophagy and other proteolytic pathways. Its significance extends beyond basic biology into clinical implications, particularly concerning neurodegenerative diseases where protein aggregation poses significant challenges. Continued research into KFERQ-related mechanisms may provide insights into novel therapeutic strategies aimed at enhancing cellular clearance systems.
Properties
Molecular Formula |
C31H50N10O9 |
---|---|
Molecular Weight |
706.8 g/mol |
IUPAC Name |
(2S)-5-amino-2-[[(2S)-2-[[(2S)-4-carboxy-2-[[(2S)-2-[[(2S)-2,6-diaminohexanoyl]amino]-3-phenylpropanoyl]amino]butanoyl]amino]-5-(diaminomethylideneamino)pentanoyl]amino]-5-oxopentanoic acid |
InChI |
InChI=1S/C31H50N10O9/c32-15-5-4-9-19(33)26(45)41-23(17-18-7-2-1-3-8-18)29(48)39-21(12-14-25(43)44)28(47)38-20(10-6-16-37-31(35)36)27(46)40-22(30(49)50)11-13-24(34)42/h1-3,7-8,19-23H,4-6,9-17,32-33H2,(H2,34,42)(H,38,47)(H,39,48)(H,40,46)(H,41,45)(H,43,44)(H,49,50)(H4,35,36,37)/t19-,20-,21-,22-,23-/m0/s1 |
InChI Key |
KIMKBQNKPBJGBS-VUBDRERZSA-N |
Isomeric SMILES |
C1=CC=C(C=C1)C[C@@H](C(=O)N[C@@H](CCC(=O)O)C(=O)N[C@@H](CCCN=C(N)N)C(=O)N[C@@H](CCC(=O)N)C(=O)O)NC(=O)[C@H](CCCCN)N |
Canonical SMILES |
C1=CC=C(C=C1)CC(C(=O)NC(CCC(=O)O)C(=O)NC(CCCN=C(N)N)C(=O)NC(CCC(=O)N)C(=O)O)NC(=O)C(CCCCN)N |
Origin of Product |
United States |
Disclaimer and Information on In-Vitro Research Products
Please be aware that all articles and product information presented on BenchChem are intended solely for informational purposes. The products available for purchase on BenchChem are specifically designed for in-vitro studies, which are conducted outside of living organisms. In-vitro studies, derived from the Latin term "in glass," involve experiments performed in controlled laboratory settings using cells or tissues. It is important to note that these products are not categorized as medicines or drugs, and they have not received approval from the FDA for the prevention, treatment, or cure of any medical condition, ailment, or disease. We must emphasize that any form of bodily introduction of these products into humans or animals is strictly prohibited by law. It is essential to adhere to these guidelines to ensure compliance with legal and ethical standards in research and experimentation.