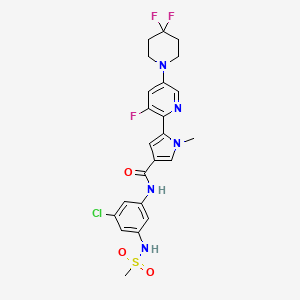
Dhx9-IN-11
- Click on QUICK INQUIRY to receive a quote from our team of experts.
- With the quality product at a COMPETITIVE price, you can focus more on your research.
Overview
Description
Dhx9-IN-11 is a small molecule inhibitor targeting the DEAH-box helicase 9 (DHX9), an ATP-independent RNA helicase. DHX9 plays a crucial role in various cellular processes, including replication, transcription, translation, RNA splicing, and RNA processing. Overexpression of DHX9 has been observed in multiple cancer types, making it an attractive target for oncology drug discovery .
Preparation Methods
Synthetic Routes and Reaction Conditions
The synthesis of Dhx9-IN-11 involves multiple steps, including the preparation of key intermediates and their subsequent coupling reactions. The specific synthetic routes and reaction conditions are proprietary and not publicly disclosed in detail. general synthetic methods for small molecule inhibitors typically involve:
Formation of Core Structure: This step involves the construction of the core scaffold of the molecule through various organic reactions such as cyclization, condensation, or coupling reactions.
Functional Group Modifications: Introduction of functional groups to enhance the molecule’s binding affinity and selectivity towards DHX9. This may involve reactions like alkylation, acylation, or halogenation.
Purification and Characterization: The final compound is purified using techniques like chromatography and characterized using spectroscopic methods such as NMR and mass spectrometry.
Industrial Production Methods
Industrial production of this compound would involve scaling up the laboratory synthesis to a larger scale, ensuring consistency and purity of the final product. This typically requires optimization of reaction conditions, use of high-throughput reactors, and stringent quality control measures.
Chemical Reactions Analysis
Types of Reactions
Dhx9-IN-11 can undergo various chemical reactions, including:
Oxidation: Introduction of oxygen atoms into the molecule, potentially altering its activity.
Reduction: Removal of oxygen atoms or addition of hydrogen atoms, which can modify the compound’s properties.
Substitution: Replacement of one functional group with another, which can be used to modify the molecule’s binding affinity and selectivity.
Common Reagents and Conditions
Common reagents used in these reactions include oxidizing agents like potassium permanganate, reducing agents like sodium borohydride, and various nucleophiles and electrophiles for substitution reactions. Reaction conditions such as temperature, solvent, and pH are optimized to achieve the desired transformations.
Major Products
The major products formed from these reactions depend on the specific modifications made to the this compound molecule. These products are typically characterized and evaluated for their biological activity and selectivity towards DHX9.
Scientific Research Applications
Dhx9-IN-11 has several scientific research applications, particularly in the fields of chemistry, biology, medicine, and industry:
Chemistry: Used as a tool compound to study the role of DHX9 in various biochemical pathways and to develop new synthetic methodologies.
Biology: Employed in research to understand the molecular mechanisms of DHX9 in cellular processes such as replication, transcription, and RNA processing.
Industry: Utilized in drug discovery programs to develop new inhibitors targeting DHX9 and related helicases.
Mechanism of Action
Dhx9-IN-11 exerts its effects by binding to the DHX9 helicase, inhibiting its ATPase activity and preventing the unwinding of double-stranded RNA and DNA. This inhibition leads to the accumulation of RNA/DNA secondary structures such as R-loops and circular RNA, inducing replication stress and ultimately causing cell cycle arrest and apoptosis in cancer cells . The molecular targets and pathways involved include the RNA splicing machinery, DNA replication origins, and various signaling pathways related to genomic stability .
Comparison with Similar Compounds
Similar Compounds
Several other small molecule inhibitors target DHX9 and related helicases, including:
Dhx9-IN-2: Another DHX9 inhibitor with similar binding properties.
Rifabutin: An antibiotic that also exhibits inhibitory activity against DHX9.
Uniqueness of Dhx9-IN-11
This compound is unique in its high selectivity and potency towards DHX9, making it a valuable tool for studying the biological functions of this helicase and a promising candidate for therapeutic development. Its ability to induce replication stress and apoptosis specifically in cancer cells with overexpressed DHX9 highlights its potential as a targeted cancer therapy .
Properties
Molecular Formula |
C23H23ClF3N5O3S |
---|---|
Molecular Weight |
542.0 g/mol |
IUPAC Name |
N-[3-chloro-5-(methanesulfonamido)phenyl]-5-[5-(4,4-difluoropiperidin-1-yl)-3-fluoropyridin-2-yl]-1-methylpyrrole-3-carboxamide |
InChI |
InChI=1S/C23H23ClF3N5O3S/c1-31-13-14(22(33)29-16-8-15(24)9-17(10-16)30-36(2,34)35)7-20(31)21-19(25)11-18(12-28-21)32-5-3-23(26,27)4-6-32/h7-13,30H,3-6H2,1-2H3,(H,29,33) |
InChI Key |
AJOMVXCUXNVLIA-UHFFFAOYSA-N |
Canonical SMILES |
CN1C=C(C=C1C2=C(C=C(C=N2)N3CCC(CC3)(F)F)F)C(=O)NC4=CC(=CC(=C4)Cl)NS(=O)(=O)C |
Origin of Product |
United States |
Disclaimer and Information on In-Vitro Research Products
Please be aware that all articles and product information presented on BenchChem are intended solely for informational purposes. The products available for purchase on BenchChem are specifically designed for in-vitro studies, which are conducted outside of living organisms. In-vitro studies, derived from the Latin term "in glass," involve experiments performed in controlled laboratory settings using cells or tissues. It is important to note that these products are not categorized as medicines or drugs, and they have not received approval from the FDA for the prevention, treatment, or cure of any medical condition, ailment, or disease. We must emphasize that any form of bodily introduction of these products into humans or animals is strictly prohibited by law. It is essential to adhere to these guidelines to ensure compliance with legal and ethical standards in research and experimentation.