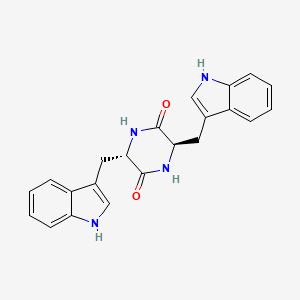
fellutanine A
Overview
Description
Fellutanine A is a bio-active diketopiperazine alkaloid isolated from the cultures of the fungus Penicillium fellutanum. It belongs to a class of naturally occurring 2,5-diketopiperazines. Initially, this compound was thought to be based on the “trans” cyclic dipeptide cyclo (L-tryptophan-D-tryptophan) but was later shown to be based on the “cis” cyclic dipeptide cyclo (L-tryptophan-L-tryptophan) .
Preparation Methods
Synthetic Routes and Reaction Conditions
The synthesis of fellutanine A involves the cyclization of L-tryptophan derivatives. The reaction typically requires the use of a coupling reagent such as dicyclohexylcarbodiimide (DCC) to facilitate the formation of the diketopiperazine ring. The reaction conditions often include anhydrous solvents and inert atmosphere to prevent unwanted side reactions .
Industrial Production Methods
Industrial production of this compound can be achieved through fermentation processes using Penicillium fellutanum cultures. The fermentation broth is extracted with organic solvents, and the compound is purified using chromatographic techniques .
Chemical Reactions Analysis
Types of Reactions
Fellutanine A undergoes various chemical reactions, including:
Oxidation: this compound can be oxidized to form corresponding diketopiperazine derivatives.
Reduction: Reduction reactions can modify the indole rings present in this compound.
Substitution: Substitution reactions can occur at the indole nitrogen or the diketopiperazine ring.
Common Reagents and Conditions
Oxidation: Common oxidizing agents include potassium permanganate and hydrogen peroxide.
Reduction: Reducing agents such as sodium borohydride and lithium aluminum hydride are used.
Substitution: Reagents like alkyl halides and acyl chlorides are employed for substitution reactions.
Major Products Formed
The major products formed from these reactions include various substituted and oxidized diketopiperazine derivatives, which can have different biological activities .
Scientific Research Applications
Fellutanine A has several scientific research applications:
Chemistry: It is used as a model compound for studying diketopiperazine chemistry and synthesis.
Biology: this compound exhibits antibacterial activity and is used in studies related to microbial resistance.
Medicine: Research is ongoing to explore its potential as an anticancer agent due to its bioactive properties.
Industry: It is used in the development of new pharmaceuticals and agrochemicals.
Mechanism of Action
Fellutanine A exerts its effects by interacting with specific molecular targets and pathways. It binds to bacterial enzymes, inhibiting their activity and leading to antibacterial effects. The compound also interacts with cellular pathways involved in cell cycle regulation, which contributes to its potential anticancer properties .
Comparison with Similar Compounds
Similar Compounds
Fellutanine B, C, and D: These are analogues of fellutanine A, differing in their structural modifications.
Cyclo (L-tryptophan-L-tryptophan): The non-annulated analogue of this compound.
Sartoryglabramides A and B: New cyclotetrapeptides isolated from marine fungi, structurally related to this compound.
Uniqueness
This compound is unique due to its specific diketopiperazine structure and its bioactivity profile. Unlike some of its analogues, this compound is not cytotoxic, making it a promising candidate for further research and development .
Properties
IUPAC Name |
(3S,6R)-3,6-bis(1H-indol-3-ylmethyl)piperazine-2,5-dione | |
---|---|---|
Source | PubChem | |
URL | https://pubchem.ncbi.nlm.nih.gov | |
Description | Data deposited in or computed by PubChem | |
InChI |
InChI=1S/C22H20N4O2/c27-21-19(9-13-11-23-17-7-3-1-5-15(13)17)25-22(28)20(26-21)10-14-12-24-18-8-4-2-6-16(14)18/h1-8,11-12,19-20,23-24H,9-10H2,(H,25,28)(H,26,27)/t19-,20+ | |
Source | PubChem | |
URL | https://pubchem.ncbi.nlm.nih.gov | |
Description | Data deposited in or computed by PubChem | |
InChI Key |
DNHODRZUCGXYKU-BGYRXZFFSA-N | |
Source | PubChem | |
URL | https://pubchem.ncbi.nlm.nih.gov | |
Description | Data deposited in or computed by PubChem | |
Canonical SMILES |
C1=CC=C2C(=C1)C(=CN2)CC3C(=O)NC(C(=O)N3)CC4=CNC5=CC=CC=C54 | |
Source | PubChem | |
URL | https://pubchem.ncbi.nlm.nih.gov | |
Description | Data deposited in or computed by PubChem | |
Isomeric SMILES |
C1=CC=C2C(=C1)C(=CN2)C[C@@H]3C(=O)N[C@H](C(=O)N3)CC4=CNC5=CC=CC=C54 | |
Source | PubChem | |
URL | https://pubchem.ncbi.nlm.nih.gov | |
Description | Data deposited in or computed by PubChem | |
Molecular Formula |
C22H20N4O2 | |
Source | PubChem | |
URL | https://pubchem.ncbi.nlm.nih.gov | |
Description | Data deposited in or computed by PubChem | |
DSSTOX Substance ID |
DTXSID10424930 | |
Record name | cyclo(Trp-Trp) | |
Source | EPA DSSTox | |
URL | https://comptox.epa.gov/dashboard/DTXSID10424930 | |
Description | DSSTox provides a high quality public chemistry resource for supporting improved predictive toxicology. | |
Molecular Weight |
372.4 g/mol | |
Source | PubChem | |
URL | https://pubchem.ncbi.nlm.nih.gov | |
Description | Data deposited in or computed by PubChem | |
CAS No. |
175414-35-4 | |
Record name | cyclo(Trp-Trp) | |
Source | EPA DSSTox | |
URL | https://comptox.epa.gov/dashboard/DTXSID10424930 | |
Description | DSSTox provides a high quality public chemistry resource for supporting improved predictive toxicology. | |
Retrosynthesis Analysis
AI-Powered Synthesis Planning: Our tool employs the Template_relevance Pistachio, Template_relevance Bkms_metabolic, Template_relevance Pistachio_ringbreaker, Template_relevance Reaxys, Template_relevance Reaxys_biocatalysis model, leveraging a vast database of chemical reactions to predict feasible synthetic routes.
One-Step Synthesis Focus: Specifically designed for one-step synthesis, it provides concise and direct routes for your target compounds, streamlining the synthesis process.
Accurate Predictions: Utilizing the extensive PISTACHIO, BKMS_METABOLIC, PISTACHIO_RINGBREAKER, REAXYS, REAXYS_BIOCATALYSIS database, our tool offers high-accuracy predictions, reflecting the latest in chemical research and data.
Strategy Settings
Precursor scoring | Relevance Heuristic |
---|---|
Min. plausibility | 0.01 |
Model | Template_relevance |
Template Set | Pistachio/Bkms_metabolic/Pistachio_ringbreaker/Reaxys/Reaxys_biocatalysis |
Top-N result to add to graph | 6 |
Feasible Synthetic Routes
Q1: What is the structure of fellutanine and its key physicochemical properties?
A1: Fellutanine is a cyclic dipeptide characterized by a diketopiperazine core structure formed by the condensation of two tryptophan molecules.
- Spectroscopic Data: Detailed spectroscopic characterization, including 1D and 2D NMR (Nuclear Magnetic Resonance) and HR-ESI-MS (High-Resolution Electrospray Ionization Mass Spectrometry), has been instrumental in elucidating the structure of fellutanine and its derivatives. [, , ]
Q2: Which fungal species are known to produce fellutanine?
A2: Fellutanine has been identified as a secondary metabolite in several fungal species, including:
- Penicillium piscarium [, ]
- Penicillium fellutanum []
- Neosartorya fischeri [, ]
- Aspergillus candidus []
Q3: Does the type of culture medium affect fellutanine production?
A: Research suggests that the production of fellutanine and related alkaloids can be influenced by the culture medium. For instance, Penicillium species have shown the ability to produce similar alkaloid profiles when grown on both wheat grain and a synthetic medium. []
Q4: Has fellutanine shown any promising biological activities?
A: While fellutanine itself has not been extensively studied for bioactivity, a related compound, fellutanine C, isolated from Penicillium setosum, exhibited significant cytotoxic activity against the human leukemia cell line Kasumi-1. [] This finding suggests potential anti-cancer properties within this class of compounds.
Q5: Are there any known enzymes involved in fellutanine biosynthesis?
A: Yes, a reverse prenyltransferase enzyme named CdpC2PT, isolated from Neosartorya fischeri, has been implicated in the biosynthesis of fellutanine. This enzyme catalyzes the reverse C2-prenylation of tryptophan-containing cyclic dipeptides, a crucial step in the proposed fellutanine biosynthetic pathway. [, ] Interestingly, CdpC2PT shows a distinct substrate preference compared to other known reverse C2-prenyltransferases, favoring substrates like (S)-benzodiazepinedinone and cyclo-L-Trp-L-Trp. [, ]
Q6: What analytical techniques are commonly used to identify and quantify fellutanine?
A6: Various chromatographic and spectroscopic methods are employed for the isolation, identification, and quantification of fellutanine. These include:
- High-Performance Liquid Chromatography (HPLC): Used for separating fellutanine from other compounds in complex mixtures. []
- Nuclear Magnetic Resonance (NMR) Spectroscopy: Provides detailed structural information about fellutanine. [, , ]
- Mass Spectrometry (MS): Determines the molecular weight and fragmentation pattern of fellutanine, aiding in its identification. [, , ]
Disclaimer and Information on In-Vitro Research Products
Please be aware that all articles and product information presented on BenchChem are intended solely for informational purposes. The products available for purchase on BenchChem are specifically designed for in-vitro studies, which are conducted outside of living organisms. In-vitro studies, derived from the Latin term "in glass," involve experiments performed in controlled laboratory settings using cells or tissues. It is important to note that these products are not categorized as medicines or drugs, and they have not received approval from the FDA for the prevention, treatment, or cure of any medical condition, ailment, or disease. We must emphasize that any form of bodily introduction of these products into humans or animals is strictly prohibited by law. It is essential to adhere to these guidelines to ensure compliance with legal and ethical standards in research and experimentation.