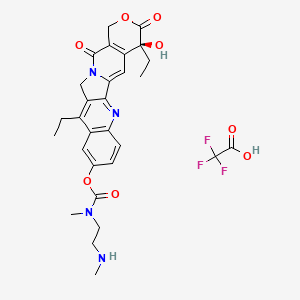
SN-38-CO-Dmeda tfa
- Click on QUICK INQUIRY to receive a quote from our team of experts.
- With the quality product at a COMPETITIVE price, you can focus more on your research.
Overview
Description
SN-38-CO-DMEDA TFA (CAS: 1224601-17-5) is a critical intermediate in synthesizing antibody-drug conjugates (ADCs), specifically for the ADC candidate LND1035 . Derived from SN-38, the active metabolite of irinotecan, this compound is modified with a carbamate-linked DMEDA (dimethylethylenediamine) group and trifluoroacetic acid (TFA) as a counterion. Its primary role is to enable conjugation of the cytotoxic payload (SN-38) to monoclonal antibodies via a stable linker, ensuring targeted delivery to cancer cells while minimizing systemic toxicity .
Key properties include:
- Molecular formula: Not explicitly stated in evidence but inferred to include SN-38 (C₂₂H₂₀N₂O₅) combined with DMEDA (C₄H₁₂N₂) and TFA (C₂HF₃O₂).
- Application: ADC development, particularly for oncology therapeutics.
- Biological relevance: SN-38 inhibits topoisomerase I, inducing DNA damage and apoptosis in cancer cells .
Preparation Methods
Synthetic Routes and Reaction Conditions
The synthesis of SN-38-CO-Dmeda trifluoroacetate involves multiple steps. The starting material, SN-38, undergoes a series of chemical reactions to introduce the dimethyl ethylenediamine (Dmeda) moiety and the trifluoroacetate group. The reaction conditions typically involve the use of organic solvents, catalysts, and controlled temperatures to ensure the desired product is obtained with high purity .
Industrial Production Methods
Industrial production of SN-38-CO-Dmeda trifluoroacetate follows similar synthetic routes but on a larger scale. The process is optimized for efficiency, yield, and cost-effectiveness. This involves the use of large reactors, automated systems for monitoring and controlling reaction conditions, and purification techniques such as crystallization and chromatography to achieve the desired purity levels .
Chemical Reactions Analysis
Types of Reactions
SN-38-CO-Dmeda trifluoroacetate undergoes various chemical reactions, including:
Oxidation: The compound can be oxidized under specific conditions to form oxidized derivatives.
Reduction: Reduction reactions can be performed to modify the functional groups present in the compound.
Substitution: The compound can undergo substitution reactions where one functional group is replaced by another.
Common Reagents and Conditions
Common reagents used in these reactions include oxidizing agents like hydrogen peroxide, reducing agents such as sodium borohydride, and various nucleophiles for substitution reactions. The reaction conditions, including temperature, solvent, and pH, are carefully controlled to achieve the desired outcomes .
Major Products Formed
The major products formed from these reactions depend on the specific reagents and conditions used. For example, oxidation may yield oxidized derivatives, while substitution reactions can introduce new functional groups into the molecule .
Scientific Research Applications
SN-38-CO-Dmeda trifluoroacetate has several scientific research applications:
Chemistry: It is used as an intermediate in the synthesis of complex molecules, particularly in the development of new drugs.
Biology: The compound is studied for its interactions with biological molecules and its potential effects on cellular processes.
Medicine: SN-38-CO-Dmeda trifluoroacetate is crucial in the development of antibody-drug conjugates, which are used in targeted cancer therapies.
Industry: The compound is used in the production of pharmaceuticals and other chemical products
Mechanism of Action
The mechanism of action of SN-38-CO-Dmeda trifluoroacetate involves its role as an intermediate in the synthesis of antibody-drug conjugates. These conjugates target specific antigens on cancer cells, delivering the cytotoxic SN-38 directly to the tumor site. This targeted delivery enhances the efficacy of the drug while minimizing side effects. The molecular targets and pathways involved include the binding of the antibody to the antigen and the subsequent internalization and release of SN-38 within the cancer cells .
Comparison with Similar Compounds
SN-38 (7-Ethyl-10-hydroxycamptothecin)
CAS : 86639-52-3
Molecular formula : C₂₂H₂₀N₂O₅
Molecular weight : 392.4 g/mol
Key differences :
- SN-38 is the free cytotoxic agent, whereas SN-38-CO-DMEDA TFA is a modified derivative designed for ADC conjugation.
- SN-38 has poor aqueous solubility (25 mg/mL in DMSO), limiting its direct therapeutic use. This compound’s DMEDA linker and TFA counterion likely enhance solubility and stability during ADC synthesis .
- Biological activity: SN-38 directly targets topoisomerase I with an IC₅₀ in the nanomolar range, while this compound requires linker cleavage in target cells to release active SN-38 .
Glu-SN38 (Glucose-Conjugated SN-38)
Synthesis : SN-38 is conjugated to glucose azides via copper-catalyzed click chemistry, improving solubility and tumor-targeting specificity .
Key differences :
- Linker type : Glu-SN38 uses a hydrophilic glucose-based linker for passive targeting via enhanced permeability and retention (EPR) effects. This compound employs a DMEDA linker, which may offer pH-dependent cleavage or improved antibody compatibility .
- Stability : Glu-SN38’s glycosidic bonds may hydrolyze prematurely in circulation, whereas this compound’s carbamate linkage is more stable, ensuring payload release only in target cells .
Other ADC Payload-Linker Intermediates
Key distinctions :
- Linker stability: this compound’s carbamate linker is less prone to nonspecific cleavage than maleimide-based linkers, reducing off-target toxicity .
- Release mechanism : Unlike protease-cleavable linkers (e.g., Val-Cit), DMEDA may rely on intracellular pH or esterase activity, offering tunable release kinetics .
Research Implications and Challenges
- Advantages of this compound :
- Enhanced stability and solubility compared to unmodified SN-36.
- Compatibility with diverse antibody conjugation strategies (e.g., lysine or cysteine coupling).
- Challenges: Potential immunogenicity of the DMEDA linker. Limited data on in vivo pharmacokinetics compared to established linkers (e.g., Val-Cit) .
Biological Activity
SN-38: The Active Metabolite
SN-38 is the active metabolite of irinotecan, a topoisomerase I inhibitor used in cancer treatment. It exhibits 1000-fold greater cytotoxicity compared to its parent drug . The biological activity of SN-38 includes:
- Inhibition of DNA and RNA synthesis
- Induction of double-stranded DNA breaks during the mitotic S-phase
- Potent anti-cancer effects against various tumor types
Biological Activity in ADCs
When incorporated into ADCs, SN-38 demonstrates enhanced therapeutic potential:
- Targeted Delivery : Conjugation to antibodies allows for specific targeting of cancer cells expressing certain antigens, such as TROP-2 .
- Improved Efficacy : ADCs incorporating SN-38 have shown enhanced activity compared to irinotecan or free SN-38 in various cancer models .
- Bystander Effect : The use of moderately stable linkers permits the release of SN-38 in the acidic tumor microenvironment, potentially affecting neighboring cancer cells .
- Protection from Inactivation : Conjugation to antibodies protects SN-38 from glucuronidation, a process that contributes to toxicity in irinotecan therapy .
Case Study: Sacituzumab Govitecan
One prominent example of an SN-38-based ADC is sacituzumab govitecan, which targets TROP-2. This ADC has demonstrated significant clinical activity:
Cancer Type | Response Rate | Median PFS |
---|---|---|
Triple-negative breast cancer | 33.3% | 5.5 months |
Small cell lung cancer | 17% | 5.2 months |
Urothelial cancer | 31% | 7.3 months |
Note: PFS = Progression-Free Survival
These results led to the FDA granting Breakthrough Therapy status for sacituzumab govitecan in advanced, refractory, metastatic triple-negative breast cancer .
Research Findings
- Enhanced Cellular Uptake : Studies have shown that SN-38-based ADCs achieve improved drug uptake by targeted cancer cells due to their high drug-to-antibody ratio (7-8:1) and repeated dosing schedules .
- Lactone Ring Stabilization : The conjugation method used in some SN-38 ADCs stabilizes the lactone ring of the SN-38 molecule, which is crucial for its biological activity .
- Reduced Toxicity : By protecting SN-38 from glucuronidation while conjugated, these ADCs potentially reduce the risk of severe diarrhea associated with irinotecan therapy .
- Bystander Effect : Research suggests that the local release of SN-38 from ADCs in the tumor microenvironment may contribute to a bystander effect, affecting nearby cancer cells not directly targeted by the antibody .
- Efficacy in Drug-Resistant Models : SN-38-based ADCs have shown activity in cancer models resistant to other therapies, potentially due to their unique mechanism of action and targeted delivery .
Q & A
Basic Research Questions
Q. What is the mechanism of action of SN-38-CO-Dmeda TFA in antibody-drug conjugates (ADCs), and how does its structure influence cytotoxicity?
this compound is an ADC comprising SN-38 (a topoisomerase I inhibitor derived from irinotecan) conjugated to an antibody via a cleavable linker. SN-38 induces DNA damage by stabilizing topoisomerase I-DNA complexes, leading to replication fork collapse and apoptosis . The linker (CL2E) ensures stability in circulation while enabling intracellular release of SN-38 via enzymatic cleavage in target cells, minimizing off-target toxicity . Structural optimization of the linker impacts payload release kinetics and ADC stability, which can be assessed using in vitro serum stability assays (e.g., monitoring SN-38 release via HPLC) .
Q. How do researchers validate the stability of this compound in biological matrices during pharmacokinetic studies?
Stability validation involves incubating the ADC in human or murine serum at 37°C and quantifying intact conjugate vs. free SN-38 over time using reversed-phase HPLC or LC-MS/MS. For example, studies show >95% of SN-38 remains bound to the antibody in serum over 72 hours, with a release half-life of ~17.5 hours . Concurrently, glucuronidation of free SN-38 (a detoxification pathway) is suppressed when the drug is conjugated, as shown by in vitro assays with UDP-glucuronosyltransferase (UGT) isoforms .
Q. What in vitro models are appropriate for assessing the antitumor efficacy of this compound?
Cell viability assays (e.g., MTT or CellTiter-Glo) in tumor cell lines expressing the target antigen are standard. For example, in colorectal cancer models (e.g., SW620), combination studies with SN-38 and gene-silencing agents (e.g., PLK1 shRNA) enhance apoptosis, as quantified via flow cytometry (Annexin V/PI staining) . Co-culture systems with stromal cells can further evaluate tumor microenvironment effects on ADC efficacy .
Advanced Research Questions
Q. How should researchers design experiments to quantify intratumoral SN-38 distribution following this compound administration?
Use fluorescence imaging or microdialysis coupled with HPLC for spatial and temporal resolution. In xenograft models (e.g., U-87MG glioblastoma), polymeric depots releasing SN-38 enable 3D reconstruction of drug distribution via intrinsic fluorescence . Microdialysis probes calibrated using the zero-flow rate (ZFR) method achieve recovery rates >70% for SN-38 in tumor homogenates, ensuring accurate measurement of free vs. protein-bound drug fractions .
Q. What methodological considerations are critical when reconciling discrepancies in SN-38 pharmacokinetic data across studies?
Key factors include:
- Sample processing : Immediate stabilization of plasma/tissue samples to prevent ex vivo SN-38 release or degradation .
- Analytical variability : Cross-validate HPLC/MS methods between labs using shared reference standards .
- Model differences : Tumor type (e.g., LS174T vs. GW-39) and ADC dosing regimens significantly affect AUC ratios (SN-38:tumor vs. blood) . Use mixed-effects statistical models to account for inter-study variability .
Q. How can researchers optimize this compound delivery to overcome drug resistance mediated by efflux transporters (e.g., BCRP/ABCG2)?
Co-administration with efflux pump inhibitors (e.g., Ko143) or ADC redesign to bypass transporter recognition are strategies. For instance, SN-38 analogs like CH-0793076 TFA retain activity in BCRP-overexpressing cells, as shown in cytotoxicity assays (IC50 = 2.3 μM) . Alternatively, liposomal encapsulation of SN-38 (e.g., S-PEG liposomes) enhances tumor accumulation and reduces glucuronidation, as demonstrated in Lewis lung carcinoma models .
Q. What experimental approaches are recommended to assess off-target toxicity of this compound in preclinical models?
- Histopathology : H&E and Ki67/Caspase-3 staining in non-target tissues (e.g., intestinal explants) to evaluate apoptosis and proliferation .
- Toxicokinetics : Compare SN-38 levels in target tumors vs. sensitive organs (e.g., liver, intestines) using AUC analysis. IMMU-132 (anti-Trop-2 ADC) reduces intestinal SN-38 exposure by 9-fold compared to irinotecan .
- Immune profiling : Flow cytometry of splenic lymphocytes to assess immune cell depletion, a common ADC toxicity .
Q. Methodological Best Practices
- Data Presentation : Organize results around specific research questions (e.g., "How does linker chemistry affect ADC stability?") using tables with descriptive titles (e.g., "Table 1: SN-38 Release Kinetics in Serum") and statistical annotations (mean ± SD, p-values) .
- Replication : Include positive controls (e.g., irinotecan-treated samples) and replicate experiments across ≥3 independent runs to ensure robustness .
- Troubleshooting : If ADC stability assays show unexpected SN-38 release, check for residual protease activity in serum batches or linker degradation under storage conditions .
Properties
Molecular Formula |
C29H31F3N4O8 |
---|---|
Molecular Weight |
620.6 g/mol |
IUPAC Name |
[(19S)-10,19-diethyl-19-hydroxy-14,18-dioxo-17-oxa-3,13-diazapentacyclo[11.8.0.02,11.04,9.015,20]henicosa-1(21),2,4(9),5,7,10,15(20)-heptaen-7-yl] N-methyl-N-[2-(methylamino)ethyl]carbamate;2,2,2-trifluoroacetic acid |
InChI |
InChI=1S/C27H30N4O6.C2HF3O2/c1-5-16-17-11-15(37-26(34)30(4)10-9-28-3)7-8-21(17)29-23-18(16)13-31-22(23)12-20-19(24(31)32)14-36-25(33)27(20,35)6-2;3-2(4,5)1(6)7/h7-8,11-12,28,35H,5-6,9-10,13-14H2,1-4H3;(H,6,7)/t27-;/m0./s1 |
InChI Key |
OPVMUGFXGHURAV-YCBFMBTMSA-N |
Isomeric SMILES |
CCC1=C2CN3C(=CC4=C(C3=O)COC(=O)[C@@]4(CC)O)C2=NC5=C1C=C(C=C5)OC(=O)N(C)CCNC.C(=O)(C(F)(F)F)O |
Canonical SMILES |
CCC1=C2CN3C(=CC4=C(C3=O)COC(=O)C4(CC)O)C2=NC5=C1C=C(C=C5)OC(=O)N(C)CCNC.C(=O)(C(F)(F)F)O |
Origin of Product |
United States |
Disclaimer and Information on In-Vitro Research Products
Please be aware that all articles and product information presented on BenchChem are intended solely for informational purposes. The products available for purchase on BenchChem are specifically designed for in-vitro studies, which are conducted outside of living organisms. In-vitro studies, derived from the Latin term "in glass," involve experiments performed in controlled laboratory settings using cells or tissues. It is important to note that these products are not categorized as medicines or drugs, and they have not received approval from the FDA for the prevention, treatment, or cure of any medical condition, ailment, or disease. We must emphasize that any form of bodily introduction of these products into humans or animals is strictly prohibited by law. It is essential to adhere to these guidelines to ensure compliance with legal and ethical standards in research and experimentation.