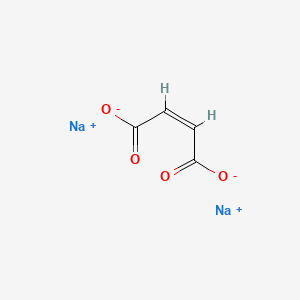
Disodium maleate
- Click on QUICK INQUIRY to receive a quote from our team of experts.
- With the quality product at a COMPETITIVE price, you can focus more on your research.
Overview
Description
Disodium maleate is an organic sodium salt that is the disodium salt of maleic acid. It has a role as an algal metabolite, a mouse metabolite and a plant metabolite. It contains a maleate(2-).
Mechanism of Action
Mode of Action
It is also used in the catalytic transfer hydrogenation of maleic acid with stoichiometric amounts of formic acid .
Biochemical Pathways
Disodium maleate is involved in the aqueous phase hydrogenation of maleic acid to succinic acid . This process is carried out in the absence of any organic solvent and using a stoichiometric amount of formic acid as a source of H2 . The reaction proceeds via catalytic transfer hydrogenation .
Result of Action
The result of the action of this compound is the transformation of maleic acid to succinic acid with negligible formation of malic acid . This transformation is possible by using relatively mild temperature (140–150 °C) and a high catalyst to maleic acid ratio .
Action Environment
The action of this compound can be influenced by environmental factors. For example, in the catalytic transfer hydrogenation of maleic acid, the reaction rate increases when using the carboxylate forms (this compound and sodium formate) instead of the acids . Additionally, the hygroscopic behavior of this compound can affect its action in atmospheric conditions .
Biochemical Analysis
Biochemical Properties
Disodium maleate plays a significant role in biochemical reactions. It interacts with enzymes, proteins, and other biomolecules, influencing their activity and function. For instance, this compound is known to interact with maleate isomerase, an enzyme that catalyzes the isomerization of maleate to fumarate . This interaction is crucial in the metabolic pathway of nicotinate metabolism in bacteria . Additionally, this compound can act as a chelating agent, binding to metal ions and affecting their availability in biochemical reactions .
Cellular Effects
This compound has various effects on different types of cells and cellular processes. It influences cell function by affecting cell signaling pathways, gene expression, and cellular metabolism. For example, this compound has been shown to inhibit the activity of certain enzymes involved in the citric acid cycle, leading to alterations in cellular energy production . Furthermore, this compound can impact gene expression by modulating the activity of transcription factors and other regulatory proteins .
Molecular Mechanism
The molecular mechanism of this compound involves its interactions with biomolecules at the molecular level. This compound can bind to enzymes, inhibiting or activating their activity. For instance, it inhibits the enzyme fumarase, which catalyzes the reversible hydration of fumarate to malate in the citric acid cycle . This inhibition can lead to an accumulation of fumarate and a decrease in malate levels, affecting cellular metabolism. Additionally, this compound can influence gene expression by binding to transcription factors and altering their activity .
Temporal Effects in Laboratory Settings
In laboratory settings, the effects of this compound can change over time. The stability and degradation of this compound can influence its long-term effects on cellular function. Studies have shown that this compound is relatively stable under standard laboratory conditions, but it can degrade over time, leading to a decrease in its effectiveness . Long-term exposure to this compound can result in changes in cellular metabolism and function, as well as potential toxic effects .
Dosage Effects in Animal Models
The effects of this compound vary with different dosages in animal models. At low doses, this compound can have beneficial effects on cellular metabolism and function. At high doses, it can cause toxic or adverse effects, such as liver and kidney damage . Studies have shown that there is a threshold dose above which the toxic effects of this compound become significant . It is important to carefully control the dosage of this compound in animal studies to avoid these adverse effects.
Metabolic Pathways
This compound is involved in several metabolic pathways, including the citric acid cycle and nicotinate metabolism. It interacts with enzymes such as fumarase and maleate isomerase, affecting their activity and the overall metabolic flux . This compound can also influence the levels of metabolites in these pathways, leading to changes in cellular energy production and other metabolic processes .
Transport and Distribution
Within cells and tissues, this compound is transported and distributed through various mechanisms. It can interact with transporters and binding proteins, affecting its localization and accumulation . For example, this compound can be transported into cells via specific transporters and then distributed to different cellular compartments . This distribution can influence its activity and function within the cell.
Subcellular Localization
The subcellular localization of this compound is crucial for its activity and function. This compound can be directed to specific compartments or organelles within the cell through targeting signals or post-translational modifications . For instance, this compound can localize to the mitochondria, where it can affect the activity of enzymes involved in the citric acid cycle . This localization is important for its role in cellular metabolism and other biochemical processes.
Properties
CAS No. |
371-47-1 |
---|---|
Molecular Formula |
C4H4NaO4 |
Molecular Weight |
139.06 g/mol |
IUPAC Name |
disodium;(Z)-but-2-enedioate |
InChI |
InChI=1S/C4H4O4.Na/c5-3(6)1-2-4(7)8;/h1-2H,(H,5,6)(H,7,8);/b2-1-; |
InChI Key |
RYDFXSRVZBYYJV-ODZAUARKSA-N |
Isomeric SMILES |
C(=C\C(=O)O)\C(=O)O.[Na] |
SMILES |
C(=CC(=O)[O-])C(=O)[O-].[Na+].[Na+] |
Canonical SMILES |
C(=CC(=O)O)C(=O)O.[Na] |
371-47-1 | |
physical_description |
Pellets or Large Crystals Solid; [Sigma-Aldrich MSDS] |
Pictograms |
Irritant |
Related CAS |
18016-19-8 |
Synonyms |
hydrogen maleate maleate maleic acid maleic acid, ammonium salt maleic acid, calcium salt maleic acid, dipotassium salt maleic acid, disodium salt maleic acid, iron salt maleic acid, monoammonium salt maleic acid, monocopper (2+) salt maleic acid, monosodium salt maleic acid, neodymium salt maleic acid, potassium salt maleic acid, sodium salt sodium maleate |
Origin of Product |
United States |
Retrosynthesis Analysis
AI-Powered Synthesis Planning: Our tool employs the Template_relevance Pistachio, Template_relevance Bkms_metabolic, Template_relevance Pistachio_ringbreaker, Template_relevance Reaxys, Template_relevance Reaxys_biocatalysis model, leveraging a vast database of chemical reactions to predict feasible synthetic routes.
One-Step Synthesis Focus: Specifically designed for one-step synthesis, it provides concise and direct routes for your target compounds, streamlining the synthesis process.
Accurate Predictions: Utilizing the extensive PISTACHIO, BKMS_METABOLIC, PISTACHIO_RINGBREAKER, REAXYS, REAXYS_BIOCATALYSIS database, our tool offers high-accuracy predictions, reflecting the latest in chemical research and data.
Strategy Settings
Precursor scoring | Relevance Heuristic |
---|---|
Min. plausibility | 0.01 |
Model | Template_relevance |
Template Set | Pistachio/Bkms_metabolic/Pistachio_ringbreaker/Reaxys/Reaxys_biocatalysis |
Top-N result to add to graph | 6 |
Feasible Synthetic Routes
Disclaimer and Information on In-Vitro Research Products
Please be aware that all articles and product information presented on BenchChem are intended solely for informational purposes. The products available for purchase on BenchChem are specifically designed for in-vitro studies, which are conducted outside of living organisms. In-vitro studies, derived from the Latin term "in glass," involve experiments performed in controlled laboratory settings using cells or tissues. It is important to note that these products are not categorized as medicines or drugs, and they have not received approval from the FDA for the prevention, treatment, or cure of any medical condition, ailment, or disease. We must emphasize that any form of bodily introduction of these products into humans or animals is strictly prohibited by law. It is essential to adhere to these guidelines to ensure compliance with legal and ethical standards in research and experimentation.