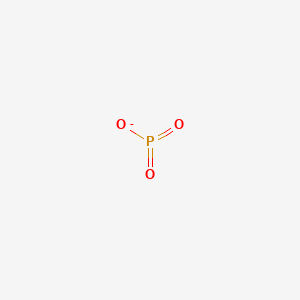
Phosphonate
Overview
Description
Phosphonates are a class of organophosphorus compounds characterized by a stable carbon-to-phosphorus (C—P) bond. These compounds are known for their resistance to biochemical, thermal, and photochemical decomposition . Phosphonates are widely used in various applications, including agriculture, medicine, and industry, due to their unique chemical properties .
Preparation Methods
Phosphonates can be synthesized through several methods, including:
Michaelis-Arbuzov Reaction: This reaction involves the conversion of trialkyl phosphites to phosphonate esters using alkyl halides.
Kabachnik-Fields Reaction: This method involves the reaction of phosphorous acid with aldehydes and amines to produce aminophosphonates.
Pudovik Reaction: This reaction involves the addition of phosphorous acid to alkenes or alkynes to form phosphonates.
Industrial Production: Industrially, phosphonates are produced by the reaction of phosphorous acid with formaldehyde and ammonia to form nitrilotris(methylenephosphonic acid).
Chemical Reactions Analysis
Key Reactions of Phosphonates
-
Hydrolysis: Phosphonate esters are susceptible to hydrolysis under both acidic and basic conditions. Cleavage of the P-C bond requires more aggressive conditions :
-
Horner–Wadsworth–Emmons (HWE) Reaction: Dialkyl-phosphonates are deprotonated to form stabilized carbanions, which react with aldehydes to produce E-alkenes and a dialkyl-phosphate .
-
Kabachnik–Fields Reaction: This three-component reaction typically involves an aldehyde or ketone, an amine, and a phosphite to produce α-aminophosphonates .
Environmental Considerations
-
Biodegradation: Research is ongoing to improve the biodegradability of phosphonates to reduce phosphorus discharge into the environment .
-
Recovery and Recycling: Efforts are being made to develop methods for the recovery and recycling of phosphorus from phosphonates .
Data Tables of Reaction Conditions and Yields
The following tables summarize research findings on various methods for synthesizing phosphonates, including reaction conditions and yields.
Table 1: Ultrasound-Assisted Kabachnik–Fields Reaction
Entry | Phosphorus source | Catalyst | Solvent | Time (min) | Yield (%) |
---|---|---|---|---|---|
1 | P(O)(OEt)3 | — | Ethyl lactate | 90 | 75 |
2 | P(O)(OEt)3 | — | Ethyl lactate/water (3:2) | 90 | 95 |
Table 2: Microwave-Assisted Synthesis of α-aminophosphonates
Entry | Phosphorus source | Catalyst (load) | Solvent | Temperature/method | Time (h) | Yield (%) |
---|---|---|---|---|---|---|
1 | H(O)P(OEt)2 | — | — | 90 °C | 6 | 51 |
2 | H(O)P(OEt)2 | SPB (5 wt%) | Ethanol | Reflux | 0.5 | 91 |
3 | P(OMe)3 | Succinic acid | — | Ambient | 5 min | 98 |
Degradation of Phosphonates
Scientific Research Applications
Phosphonates have a wide range of scientific research applications:
Mechanism of Action
Phosphonates exert their effects through various mechanisms:
Enzyme Inhibition: Phosphonates mimic the structure of phosphate esters and inhibit enzymes that utilize phosphate esters as substrates.
Molecular Targets: The primary molecular targets of phosphonates are enzymes involved in phosphate metabolism.
Pathways Involved: Phosphonates interfere with biochemical pathways that involve phosphate esters, leading to the inhibition of key metabolic processes.
Comparison with Similar Compounds
Phosphonates are unique compared to other organophosphorus compounds due to their stable C—P bond. Similar compounds include:
Phosphates: Unlike phosphonates, phosphates have a P—O bond and are more prone to hydrolysis.
Phosphinates: Phosphinates have a P—H bond and are used in similar applications but have different chemical properties.
Phosphites: Phosphites contain a P—O bond and are used as reducing agents in organic synthesis.
Phosphonates stand out due to their stability and resistance to decomposition, making them valuable in various applications .
Q & A
Q. Basic: What experimental approaches are recommended for synthesizing phosphonate derivatives with targeted biological activity?
Methodological Answer:
Synthesis of this compound derivatives requires careful selection of precursors (e.g., alkyl halides, phosphite esters) and optimization of reaction conditions (e.g., temperature, catalysts like palladium for cross-coupling reactions). Characterization involves nuclear magnetic resonance (NMR) to confirm the phosphorus-carbon bond and mass spectrometry (MS) for molecular weight validation. For biological targeting, structure-activity relationship (SAR) studies are critical: systematically vary substituents and test against relevant assays (e.g., enzyme inhibition, cellular uptake) .
Table 1: Common Techniques for this compound Synthesis & Characterization
Technique | Application | Key Considerations |
---|---|---|
³¹P NMR | Confirming this compound bond formation | Sensitive to electronic environment |
HPLC-MS | Purity assessment | Detects trace impurities in derivatives |
X-ray Diffraction | Crystal structure determination | Requires high-quality single crystals |
Q. Advanced: How can computational modeling resolve contradictions in this compound-enzyme interaction studies?
Methodological Answer:
Contradictions in experimental data (e.g., conflicting inhibition constants) may arise from variable enzyme conformations or solvent effects. Use molecular dynamics (MD) simulations to model enzyme flexibility and docking studies to predict binding affinities. Cross-validate with mutagenesis experiments: if computational predictions align with mutant enzyme activity, this supports the model. Combine with kinetic assays (e.g., stopped-flow spectroscopy) to reconcile discrepancies .
Basic: What frameworks (e.g., PICOT) are suitable for formulating this compound-related research questions?
Methodological Answer:
The PICOT framework is effective for clinical or biochemical studies:
- P opulation: Enzyme/system under study (e.g., HEPD enzyme in E. coli).
- I ntervention: this compound compound (e.g., 2-hydroxyethylthis compound).
- C omparison: Native substrate or alternative inhibitors.
- O utcome: Metrics like IC₅₀, catalytic efficiency.
- T ime: Reaction duration or longitudinal stability tests.
For environmental studies, modify to include ecological variables (e.g., soil pH, microbial diversity) .
Q. Advanced: How to optimize this compound-PNA conjugate delivery for antisense applications?
Methodological Answer:
Enhance cellular uptake by varying this compound chain length (e.g., P7 vs. P10 in PNA conjugates) and using delivery agents like cationic lipids. Validate efficacy via luciferase reporter assays and RT-PCR to quantify splice correction. EC₅₀ values (e.g., 5 nM for P10-PNA in HeLa cells) guide dosage optimization. Compare with alternative delivery methods (e.g., electroporation) to assess efficiency .
Q. Basic: What are the ethical considerations in handling this compound toxicity data?
Methodological Answer:
Ensure transparency in reporting negative results (e.g., low toxicity in certain cell lines) to avoid publication bias. Follow FAIR principles (Findable, Accessible, Interoperable, Reusable) for data sharing. Document protocols for animal or human cell line use, including institutional review board (IRB) approvals and informed consent for clinical samples .
Q. Advanced: What strategies mitigate batch-to-batch variability in this compound production?
Methodological Answer:
Implement quality control (QC) protocols:
- Analytical Consistency: Use triple-quadrupole MS for batch comparison.
- Process Controls: Monitor reaction parameters (pH, temperature) in real time.
- Statistical Analysis: Apply multivariate analysis (e.g., PCA) to identify outlier batches.
Publish detailed synthetic procedures in supplementary materials to aid reproducibility .
Q. Basic: How to design a study investigating this compound degradation in environmental samples?
Methodological Answer:
Define scope using the FLOAT method:
- F ocus: Degradation rate in soil vs. aquatic systems.
- L ink variables: Microbial activity, pH, temperature.
- O ptimize sampling intervals (e.g., 0, 7, 14 days).
- A nalyze via LC-MS/MS for degradation products.
- T imeframe: Align with grant deadlines or seasonal variations .
Q. Advanced: How to integrate this compound biosynthetic pathways into metabolic engineering workflows?
Methodological Answer:
Use genome mining to identify this compound biosynthetic gene clusters (e.g., phn operon). Employ CRISPR-Cas9 for pathway knock-in/out in host organisms (e.g., S. cerevisiae). Monitor flux via ¹³C metabolic flux analysis and compare with wild-type strains. Address enzyme promiscuity by screening homologs (e.g., HppE vs. HEPD) for improved yield .
Q. Basic: What statistical methods are appropriate for analyzing this compound efficacy data?
Methodological Answer:
For dose-response studies, use nonlinear regression to calculate IC₅₀/EC₅₀. Apply ANOVA for multi-group comparisons (e.g., this compound vs. control). Report confidence intervals and p-values with corrections for multiple testing (e.g., Bonferroni). Use R/Bioconductor packages like drc for curve fitting .
Q. Advanced: How to validate this compound-target interactions in structurally unresolved enzymes?
Methodological Answer:
Employ hydrogen-deuterium exchange mass spectrometry (HDX-MS) to map binding-induced conformational changes. Pair with site-directed mutagenesis: if a predicted binding residue mutation abolishes activity, this supports interaction. Validate with isothermal titration calorimetry (ITC) for thermodynamic profiling .
Q. Basic: How to ensure reproducibility in this compound-related research?
Methodological Answer:
Document all experimental parameters (e.g., solvent purity, instrument calibration). Use open-source platforms like GitHub for protocol sharing. Deposit raw data in repositories (e.g., Zenodo) with DOIs. Collaborate with third-party labs for independent validation .
Q. Advanced: What systems biology approaches elucidate this compound roles in microbial communities?
Methodological Answer:
Combine metagenomics (to identify this compound-utilizing taxa) and metatranscriptomics (to assess gene expression under this compound stress). Use genome-scale metabolic models (GEMs) like AGORA to simulate community-level impacts. Validate with stable isotope probing (SIP) using ¹⁵N-labeled phosphonates .
Properties
InChI |
InChI=1S/HO3P/c1-4(2)3/h(H,1,2,3)/p-1 | |
---|---|---|
Details | Computed by InChI 1.0.5 (PubChem release 2019.06.18) | |
Source | PubChem | |
URL | https://pubchem.ncbi.nlm.nih.gov | |
Description | Data deposited in or computed by PubChem | |
InChI Key |
UEZVMMHDMIWARA-UHFFFAOYSA-M | |
Details | Computed by InChI 1.0.5 (PubChem release 2019.06.18) | |
Source | PubChem | |
URL | https://pubchem.ncbi.nlm.nih.gov | |
Description | Data deposited in or computed by PubChem | |
Canonical SMILES |
[O-]P(=O)=O | |
Details | Computed by OEChem 2.1.5 (PubChem release 2019.06.18) | |
Source | PubChem | |
URL | https://pubchem.ncbi.nlm.nih.gov | |
Description | Data deposited in or computed by PubChem | |
Molecular Formula |
O3P- | |
Details | Computed by PubChem 2.1 (PubChem release 2019.06.18) | |
Source | PubChem | |
URL | https://pubchem.ncbi.nlm.nih.gov | |
Description | Data deposited in or computed by PubChem | |
DSSTOX Substance ID |
DTXSID50422536 | |
Record name | Phosphonate | |
Source | EPA DSSTox | |
URL | https://comptox.epa.gov/dashboard/DTXSID50422536 | |
Description | DSSTox provides a high quality public chemistry resource for supporting improved predictive toxicology. | |
Molecular Weight |
78.972 g/mol | |
Details | Computed by PubChem 2.1 (PubChem release 2021.05.07) | |
Source | PubChem | |
URL | https://pubchem.ncbi.nlm.nih.gov | |
Description | Data deposited in or computed by PubChem | |
CAS No. |
60118-28-7 | |
Record name | Phosphonate | |
Source | EPA DSSTox | |
URL | https://comptox.epa.gov/dashboard/DTXSID50422536 | |
Description | DSSTox provides a high quality public chemistry resource for supporting improved predictive toxicology. | |
Disclaimer and Information on In-Vitro Research Products
Please be aware that all articles and product information presented on BenchChem are intended solely for informational purposes. The products available for purchase on BenchChem are specifically designed for in-vitro studies, which are conducted outside of living organisms. In-vitro studies, derived from the Latin term "in glass," involve experiments performed in controlled laboratory settings using cells or tissues. It is important to note that these products are not categorized as medicines or drugs, and they have not received approval from the FDA for the prevention, treatment, or cure of any medical condition, ailment, or disease. We must emphasize that any form of bodily introduction of these products into humans or animals is strictly prohibited by law. It is essential to adhere to these guidelines to ensure compliance with legal and ethical standards in research and experimentation.