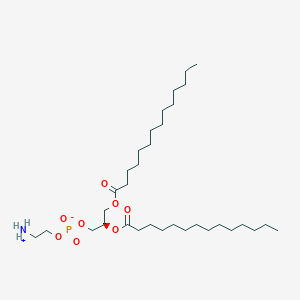
1,2-Dimyristoyl-sn-glycero-3-phosphoethanolamine
Overview
Description
1,2-Dimyristoyl-sn-glycero-3-phosphoethanolamine is a phospholipid commonly found in biological membranes. It is composed of two myristic acid chains attached to a glycerol backbone, which is further linked to a phosphoethanolamine group. This compound plays a crucial role in the structural integrity and functionality of cell membranes, contributing to membrane fluidity and the formation of lipid bilayers .
Mechanism of Action
Target of Action
1,2-Dimyristoyl-sn-glycero-3-phosphoethanolamine (DMPE) is a lipid found in the cell membranes of bacteria and human nervous tissues . It primarily targets cell membranes due to its similar phospholipid structures .
Mode of Action
DMPE interacts with its targets, the cell membranes, by integrating into the lipid bilayer. This interaction can alter the physical properties of the membrane, such as its fluidity and permeability . DMPE has been shown to enhance the constancy and in vitro antiproliferative effect of certain drugs .
Biochemical Pathways
DMPE affects the biochemical pathways related to membrane function and integrity. By integrating into the lipid bilayer, it can influence membrane-associated processes, such as signal transduction and substance transport .
Result of Action
The integration of DMPE into cell membranes can result in changes to the membrane’s physical properties, potentially affecting the function of membrane-bound proteins and the cell’s overall behavior . In some cases, DMPE has been shown to enhance the constancy and in vitro antiproliferative effect of certain drugs .
Action Environment
The action of DMPE can be influenced by various environmental factors. For instance, the presence of other lipids can affect its integration into cell membranes . Additionally, factors such as pH and temperature can influence the stability and efficacy of DMPE .
Biochemical Analysis
Biochemical Properties
1,2-Dimyristoyl-sn-glycero-3-phosphoethanolamine plays a significant role in biochemical reactions. It interacts with various enzymes, proteins, and other biomolecules. The nature of these interactions is complex and multifaceted, often involving the formation of lipid bilayers and monolayers .
Cellular Effects
The effects of this compound on cells and cellular processes are diverse. It influences cell function by impacting cell signaling pathways, gene expression, and cellular metabolism .
Molecular Mechanism
The molecular mechanism of action of this compound involves its interactions at the molecular level. It can bind to biomolecules, inhibit or activate enzymes, and induce changes in gene expression .
Temporal Effects in Laboratory Settings
Over time in laboratory settings, the effects of this compound can change. Information on the product’s stability, degradation, and long-term effects on cellular function observed in in vitro or in vivo studies is currently limited .
Metabolic Pathways
This compound is involved in several metabolic pathways. It interacts with various enzymes and cofactors, and can influence metabolic flux or metabolite levels .
Transport and Distribution
This compound is transported and distributed within cells and tissues. It can interact with transporters or binding proteins, influencing its localization or accumulation .
Subcellular Localization
The subcellular localization of this compound and its effects on activity or function are complex. It may involve targeting signals or post-translational modifications that direct it to specific compartments or organelles .
Preparation Methods
Synthetic Routes and Reaction Conditions
1,2-Dimyristoyl-sn-glycero-3-phosphoethanolamine can be synthesized through the esterification of glycerol with myristic acid, followed by the phosphorylation of the resulting diglyceride with phosphoethanolamine. The reaction typically involves the use of catalysts such as dicyclohexylcarbodiimide (DCC) and dimethylaminopyridine (DMAP) to facilitate the esterification process .
Industrial Production Methods
Industrial production of this compound involves large-scale esterification and phosphorylation processes. The use of continuous flow reactors and advanced purification techniques ensures high yield and purity of the final product .
Chemical Reactions Analysis
Types of Reactions
1,2-Dimyristoyl-sn-glycero-3-phosphoethanolamine undergoes various chemical reactions, including:
Oxidation: The myristic acid chains can be oxidized to form hydroperoxides and other oxidative products.
Reduction: The phosphoethanolamine group can be reduced to form phosphoethanolamine derivatives.
Substitution: The hydroxyl groups on the glycerol backbone can undergo substitution reactions with various reagents.
Common Reagents and Conditions
Oxidation: Common oxidizing agents include hydrogen peroxide and potassium permanganate.
Reduction: Reducing agents such as sodium borohydride and lithium aluminum hydride are used.
Substitution: Reagents like acyl chlorides and alkyl halides are employed for substitution reactions.
Major Products Formed
Oxidation: Hydroperoxides and aldehydes.
Reduction: Phosphoethanolamine derivatives.
Substitution: Various substituted glycerol derivatives.
Scientific Research Applications
1,2-Dimyristoyl-sn-glycero-3-phosphoethanolamine has a wide range of applications in scientific research:
Chemistry: Used in the synthesis of liposomes and other lipid-based structures for drug delivery systems.
Biology: Plays a role in the study of membrane dynamics and protein-lipid interactions.
Industry: Employed in the production of cosmetics and personal care products due to its emulsifying properties.
Comparison with Similar Compounds
Similar Compounds
1,2-Dimyristoyl-sn-glycero-3-phosphocholine: Similar in structure but contains a phosphocholine group instead of phosphoethanolamine.
1,2-Dimyristoyl-sn-glycero-3-phosphoglycerol: Contains a phosphoglycerol group and is used in similar applications.
1,2-Dimyristoyl-sn-glycero-3-phosphoethanolamine-N-[methoxy(polyethylene glycol)-2000]: A polyethylene glycol conjugate used in advanced drug delivery systems.
Uniqueness
This compound is unique due to its specific interaction with membrane proteins and its ability to form stable lipid bilayers. Its versatility in various scientific and industrial applications sets it apart from other similar compounds .
Properties
CAS No. |
998-07-2 |
---|---|
Molecular Formula |
C33H66NO8P |
Molecular Weight |
635.9 g/mol |
IUPAC Name |
2-azaniumylethyl [(2R)-2,3-di(tetradecanoyloxy)propyl] phosphate |
InChI |
InChI=1S/C33H66NO8P/c1-3-5-7-9-11-13-15-17-19-21-23-25-32(35)39-29-31(30-41-43(37,38)40-28-27-34)42-33(36)26-24-22-20-18-16-14-12-10-8-6-4-2/h31H,3-30,34H2,1-2H3,(H,37,38)/t31-/m1/s1 |
InChI Key |
NEZDNQCXEZDCBI-WJOKGBTCSA-N |
Isomeric SMILES |
CCCCCCCCCCCCCC(=O)OC[C@H](COP(=O)([O-])OCC[NH3+])OC(=O)CCCCCCCCCCCCC |
SMILES |
CCCCCCCCCCCCCC(=O)OCC(COP(=O)([O-])OCC[NH3+])OC(=O)CCCCCCCCCCCCC |
Canonical SMILES |
CCCCCCCCCCCCCC(=O)OCC(COP(=O)([O-])OCC[NH3+])OC(=O)CCCCCCCCCCCCC |
Appearance |
Unit:100 mgSolvent:nonePurity:98+%Physical solid |
998-07-2 | |
physical_description |
Solid |
Synonyms |
1,2-dimyristoyl-sn-glycero-3-phosphoethanolamine 1,2-dimyristoylphosphatidylethanolamine 1,2-ditetradecanoyl-sn-glycero-3-phosphoethanolamine dimyristoyl cephalin DMPE tetradecanoic acid, (1R)-1-((((2-aminoethoxy)hydroxyphosphinyl)oxy)methyl)-1,2-ethanediyl este |
Origin of Product |
United States |
Retrosynthesis Analysis
AI-Powered Synthesis Planning: Our tool employs the Template_relevance Pistachio, Template_relevance Bkms_metabolic, Template_relevance Pistachio_ringbreaker, Template_relevance Reaxys, Template_relevance Reaxys_biocatalysis model, leveraging a vast database of chemical reactions to predict feasible synthetic routes.
One-Step Synthesis Focus: Specifically designed for one-step synthesis, it provides concise and direct routes for your target compounds, streamlining the synthesis process.
Accurate Predictions: Utilizing the extensive PISTACHIO, BKMS_METABOLIC, PISTACHIO_RINGBREAKER, REAXYS, REAXYS_BIOCATALYSIS database, our tool offers high-accuracy predictions, reflecting the latest in chemical research and data.
Strategy Settings
Precursor scoring | Relevance Heuristic |
---|---|
Min. plausibility | 0.01 |
Model | Template_relevance |
Template Set | Pistachio/Bkms_metabolic/Pistachio_ringbreaker/Reaxys/Reaxys_biocatalysis |
Top-N result to add to graph | 6 |
Feasible Synthetic Routes
Q1: How does 1,2-Dimyristoyl-sn-glycero-3-phosphoethanolamine (DMPE) interact with antimicrobial peptides (AMPs)?
A: AMPs can insert themselves into DMPE bilayers to varying degrees, influencing the dynamics of the phospholipids. [] The interaction appears to be stronger with DMPE than with phosphatidylcholine (PC) lipids, leading to increased destabilization and morphological changes in DMPE vesicles upon AMP addition. []
Q2: What is the role of DMPE in bacterial membranes and its interaction with host defense peptides?
A: DMPE is a significant component of bacterial membranes. Research indicates that some host defense peptides, like Maximin H5, exhibit a higher binding affinity for DMPE-rich membranes. [] This interaction can limit the peptide's ability to lyse the bacterial membrane, potentially contributing to bacterial resistance against host defense mechanisms. []
Q3: Can the interaction between DMPE and cholesterol impact membrane properties?
A: Yes, nearest-neighbor recognition experiments suggest that cholesterol's condensing effect on phospholipid bilayers is primarily observed in the liquid-ordered phase. [] This implies that the presence of cholesterol can significantly influence the packing and organization of DMPE molecules within the membrane. []
Q4: What is the molecular formula and weight of DMPE?
A4: The molecular formula of DMPE is C36H72NO8P, and its molecular weight is 637.9 g/mol.
Q5: How does the chain length of phospholipids in a mixture affect the permeability of the resulting bilayers?
A: Studies using liposomes made from mixtures of DMPE and 1,2-distearoyl-sn-glycero-3-phosphocholine (DSPC) show that the permeability of the bilayer is affected by the composition of the lipid phases at the boundaries between liquid crystal and gel states. [] This suggests that DMPE may segregate at these boundaries, influencing the overall permeability of the membrane. []
Q6: How does DMPE behave in mixed monolayers with other phospholipids?
A: DMPE exhibits different miscibility behaviors with various phospholipids. While it shows limited miscibility with phosphoglycerol (PG)-like lipids in the fluid bilayer state, [] it displays non-ideal mixing behavior with 1,2-distearoyl-sn-glycero-3-phosphoethanolamine (DSPE) in the presence of certain diluents like 1,2-dilauroyl-sn-glycero-3-phosphocholine (DLPC). []
Q7: What is the impact of temperature on the organization of water molecules around DMPE bilayers?
A: Research using Small Angle X-ray Scattering (SAXS) and densitometry revealed that the water layer surrounding DMPE bilayers, particularly the "perturbed water" layer, expands with increasing temperature. [] This indicates a temperature-dependent interaction between DMPE and water molecules at the membrane interface. []
Q8: Does the presence of cholesterol affect the interaction between DMPE and water?
A: Studies utilizing nearest-neighbor recognition experiments with exchangeable DMPE dimers suggest that replacing water (H2O) with deuterium oxide (D2O) impacts DMPE mixing only in saturated bilayers lacking cholesterol. [] This indicates that cholesterol may influence the hydration properties of DMPE-containing membranes. []
A8: This section is not applicable as DMPE is primarily a structural lipid and not known to possess inherent catalytic properties.
Q9: How can molecular dynamics simulations be employed to understand DMPE interactions?
A: Molecular dynamics simulations can offer valuable insights into the behavior of DMPE within biological systems. For instance, simulations have been used to examine the stability of myelin basic protein (MBP) on mixed DMPC/DMPE bilayers. [] These simulations revealed that the composition of the lipid bilayer influences MBP's interaction with the membrane, providing insights into the structural dynamics of myelin. []
Q10: Can molecular modeling help understand the interaction of drug conjugates with DMPE membranes?
A: Yes, molecular modeling techniques, in conjunction with experimental methods like Langmuir monolayer studies, can provide insights into how drug conjugates interact with DMPE-based model bacterial membranes. [] This approach can reveal details about the binding modes, conformational changes, and potential impact on membrane hydration. []
Q11: How does the linkage region of sphingolipids influence their mixing with DMPE in cholesterol-rich environments?
A: Nearest-neighbor recognition studies suggest that the linkage region of sphingolipids plays a crucial role in their interaction with phospholipids like DMPE, especially in cholesterol-rich bilayers. [] The presence of cholesterol enhances the preference for homolipid association, indicating that the sphingolipid's linkage region contributes to its segregation from DMPE within the membrane. []
Q12: How does the bridging group in exchangeable DMPE dimers influence nearest-neighbor recognition (NNR) within fluid bilayers?
A: Research indicates that the length and chemical nature of the bridging group in DMPE dimers can significantly impact NNR within fluid bilayers. [] Longer bridging groups tend to enhance NNR, suggesting that they influence the packing and organization of DMPE molecules. [] Cholesterol's effect on NNR was also found to be dependent on the bridging group length. []
Q13: How stable are liposomes composed of DMPE, dihexadecyl phosphate (DCP), and cholesterol?
A: Liposomes with varying ratios of DMPE, DCP, and cholesterol have been studied for their physicochemical properties and biological activity. [] Results suggest that these liposomes are stable at physiological temperatures, making them suitable for in vivo applications. [] The addition of DCP appears to improve colloidal stability. []
A13: Specific SHE regulations are not explicitly discussed in the provided research papers.
A13: The provided research focuses mainly on the biophysical properties and interactions of DMPE rather than its pharmacokinetic and pharmacodynamic aspects.
Q14: How effective are liposomes containing DMPE in delivering therapeutic agents?
A: Studies have investigated the efficacy of DMPE-containing liposomes as delivery systems for therapeutic agents. Liposomes prepared with DMPE and DCP showed the best efficiency in an in vitro model mimicking in vivo release of bioactive substances. []
Q15: How does the presence of DMPE in bacterial membranes contribute to bacterial resistance?
A: The presence of DMPE in bacterial membranes can contribute to resistance against certain antimicrobial agents, such as host defense peptides. [] The specific interaction between DMPE and these peptides can hinder their membrane-disrupting activity, allowing bacteria to evade the immune response. []
A15: Toxicity and safety data specific to DMPE are not explicitly detailed in the provided research papers.
Q16: Can hyaluronic acid-decorated liposomes be used for targeted drug delivery to the skin?
A: Research suggests that hyaluronic acid-decorated liposomes, particularly those incorporating DMPE as a lipid anchor, hold promise for targeted drug delivery to the skin. [] The deformability of these liposomes and the extent of hyaluronic acid conjugation appear to be crucial factors influencing skin penetration. []
Q17: What is the potential of lipid nanoparticles containing DMPE for siRNA delivery to immune cells?
A: Lipid nanoparticles composed of a novel cationic lipid, DOPE, and PEG2000-DMPE have shown potential for siRNA delivery to immune cells like macrophages and dendritic cells. [] These nanoparticles demonstrated efficient siRNA encapsulation and successful gene knockdown in both in vitro and in vivo models. []
A17: Specific biomarkers and diagnostics related to DMPE are not directly addressed in the provided research papers.
Q18: What are some analytical techniques used to study DMPE-containing systems?
A18: Various techniques have been employed to characterize and study DMPE-containing systems. Some examples include:
- Langmuir monolayer studies: These studies provide insights into the interactions of DMPE with other molecules at the air-water interface. [, , ]
- Atomic force microscopy (AFM): AFM can be used to visualize the morphology and organization of DMPE molecules within model membranes. []
- Neutron reflectometry: This technique helps determine the structure and thickness of DMPE-containing layers within multilayered films. []
- Quasi-elastic neutron scattering (QENS): QENS provides information about the dynamics of DMPE molecules and associated water molecules within bilayers. []
- Polarization modulation infrared reflection-absorption spectroscopy (PM-IRRAS): PM-IRRAS offers insights into the orientation and conformation of DMPE molecules within monolayers. []
- Brewster angle microscopy (BAM): BAM visualizes the morphology and phase behavior of DMPE monolayers at the air-water interface. [, ]
- Differential scanning calorimetry (DSC): DSC measures the thermal transitions of DMPE-containing systems, providing insights into their phase behavior. [, , ]
- Dynamic light scattering (DLS): DLS measures the size and diffusion properties of DMPE-containing liposomes and vesicles. [, , , ]
- Small angle X-ray/neutron scattering (SAXS/SANS): SAXS and SANS provide structural information about DMPE-containing bilayers and vesicles. [, , ]
- Time-resolved small-angle neutron scattering (TR-SANS): TR-SANS investigates the dynamics of lipid flip-flop and exchange within DMPE-containing vesicles. [, ]
Disclaimer and Information on In-Vitro Research Products
Please be aware that all articles and product information presented on BenchChem are intended solely for informational purposes. The products available for purchase on BenchChem are specifically designed for in-vitro studies, which are conducted outside of living organisms. In-vitro studies, derived from the Latin term "in glass," involve experiments performed in controlled laboratory settings using cells or tissues. It is important to note that these products are not categorized as medicines or drugs, and they have not received approval from the FDA for the prevention, treatment, or cure of any medical condition, ailment, or disease. We must emphasize that any form of bodily introduction of these products into humans or animals is strictly prohibited by law. It is essential to adhere to these guidelines to ensure compliance with legal and ethical standards in research and experimentation.