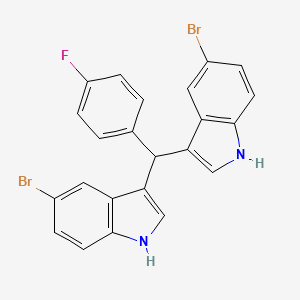
Topoisomerase I inhibitor 9
- Click on QUICK INQUIRY to receive a quote from our team of experts.
- With the quality product at a COMPETITIVE price, you can focus more on your research.
Overview
Description
Topoisomerase I inhibitor 9 is a compound that targets the enzyme topoisomerase I, which plays a crucial role in DNA replication and transcription by relieving torsional strain in the DNA helix.
Preparation Methods
Synthetic Routes and Reaction Conditions
The synthesis of topoisomerase I inhibitors typically involves multiple steps, including the formation of key intermediates and the final coupling reactions. For example, camptothecin derivatives, which are a class of topoisomerase I inhibitors, are synthesized through a series of reactions involving the formation of the lactone ring and subsequent modifications to enhance their stability and efficacy .
Industrial Production Methods
Industrial production of topoisomerase I inhibitors often involves optimizing the synthetic routes to improve yield and reduce costs. This may include the use of advanced techniques such as flow chemistry and continuous processing to scale up the production while maintaining the quality and purity of the final product .
Chemical Reactions Analysis
Types of Reactions
Topoisomerase I inhibitor 9 undergoes various chemical reactions, including:
Oxidation: This reaction can modify the functional groups on the inhibitor, potentially enhancing its activity or stability.
Reduction: Reduction reactions can be used to modify the inhibitor’s structure, potentially improving its binding affinity to topoisomerase I.
Substitution: Substitution reactions can introduce different functional groups to the inhibitor, altering its pharmacokinetic properties
Common Reagents and Conditions
Common reagents used in these reactions include oxidizing agents like hydrogen peroxide, reducing agents like sodium borohydride, and various nucleophiles for substitution reactions. The conditions for these reactions typically involve controlled temperatures and pH to ensure the desired modifications are achieved .
Major Products Formed
The major products formed from these reactions are typically modified versions of the original inhibitor, with changes in functional groups that can enhance its efficacy, stability, or pharmacokinetic properties .
Scientific Research Applications
Topoisomerase I inhibitor 9 has a wide range of scientific research applications, including:
Chemistry: Used as a tool to study DNA topology and the mechanisms of DNA replication and transcription.
Biology: Employed in research to understand the cellular processes involving DNA damage and repair.
Medicine: Investigated for its potential as an anticancer agent, particularly in targeting rapidly dividing cancer cells.
Industry: Utilized in the development of new therapeutic agents and in the study of drug resistance mechanisms .
Mechanism of Action
Topoisomerase I inhibitor 9 exerts its effects by stabilizing the topoisomerase I-DNA cleavage complex, preventing the re-ligation of the DNA strand. This leads to the accumulation of DNA breaks, ultimately causing cell death. The molecular targets involved include the topoisomerase I enzyme and the DNA itself. The pathways affected by this inhibition include DNA replication and transcription, leading to the activation of DNA damage response pathways and apoptosis .
Comparison with Similar Compounds
Topoisomerase I inhibitor 9 can be compared with other similar compounds such as:
Camptothecin: A natural product that also targets topoisomerase I but has limitations in terms of stability and solubility.
Topotecan: A semi-synthetic derivative of camptothecin with improved water solubility and reduced toxicity.
Irinotecan: Another camptothecin derivative that is widely used in cancer therapy due to its enhanced efficacy and reduced side effects .
This compound is unique in its specific modifications that enhance its binding affinity and stability, making it a promising candidate for further development as a therapeutic agent .
Properties
Molecular Formula |
C23H15Br2FN2 |
---|---|
Molecular Weight |
498.2 g/mol |
IUPAC Name |
5-bromo-3-[(5-bromo-1H-indol-3-yl)-(4-fluorophenyl)methyl]-1H-indole |
InChI |
InChI=1S/C23H15Br2FN2/c24-14-3-7-21-17(9-14)19(11-27-21)23(13-1-5-16(26)6-2-13)20-12-28-22-8-4-15(25)10-18(20)22/h1-12,23,27-28H |
InChI Key |
QHISLSGSRBIKOS-UHFFFAOYSA-N |
Canonical SMILES |
C1=CC(=CC=C1C(C2=CNC3=C2C=C(C=C3)Br)C4=CNC5=C4C=C(C=C5)Br)F |
Origin of Product |
United States |
Disclaimer and Information on In-Vitro Research Products
Please be aware that all articles and product information presented on BenchChem are intended solely for informational purposes. The products available for purchase on BenchChem are specifically designed for in-vitro studies, which are conducted outside of living organisms. In-vitro studies, derived from the Latin term "in glass," involve experiments performed in controlled laboratory settings using cells or tissues. It is important to note that these products are not categorized as medicines or drugs, and they have not received approval from the FDA for the prevention, treatment, or cure of any medical condition, ailment, or disease. We must emphasize that any form of bodily introduction of these products into humans or animals is strictly prohibited by law. It is essential to adhere to these guidelines to ensure compliance with legal and ethical standards in research and experimentation.