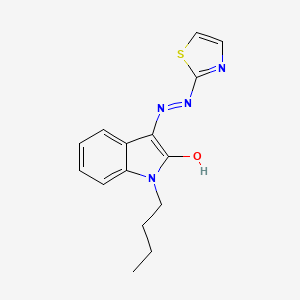
Antimicrobial agent-22
- Click on QUICK INQUIRY to receive a quote from our team of experts.
- With the quality product at a COMPETITIVE price, you can focus more on your research.
Overview
Description
Antimicrobial Agent-22 is a chemical compound known for its ability to inhibit the growth of or destroy microorganisms. It is widely used in various fields, including medicine, agriculture, and industry, to combat bacterial, fungal, and viral infections. The compound’s effectiveness and broad-spectrum activity make it a valuable tool in the fight against microbial resistance.
Preparation Methods
Synthetic Routes and Reaction Conditions: The synthesis of Antimicrobial Agent-22 typically involves a multi-step chemical process. One common method includes the reaction of a primary amine with a carboxylic acid derivative under controlled conditions. The reaction is often catalyzed by a coupling agent such as dicyclohexylcarbodiimide, and the product is purified through recrystallization or chromatography.
Industrial Production Methods: In industrial settings, the production of this compound is scaled up using continuous flow reactors. This method allows for precise control of reaction parameters, ensuring high yield and purity. The process involves the use of large-scale reactors, automated monitoring systems, and advanced purification techniques to produce the compound efficiently and cost-effectively.
Chemical Reactions Analysis
Types of Reactions: Antimicrobial Agent-22 undergoes various chemical reactions, including:
Oxidation: The compound can be oxidized using agents such as hydrogen peroxide or potassium permanganate, leading to the formation of oxidized derivatives with enhanced antimicrobial properties.
Reduction: Reduction reactions involving this compound typically use reducing agents like sodium borohydride or lithium aluminum hydride to produce reduced forms of the compound.
Substitution: The compound can undergo nucleophilic substitution reactions, where functional groups are replaced by nucleophiles such as halides or alkoxides.
Common Reagents and Conditions:
Oxidation: Hydrogen peroxide, potassium permanganate; acidic or basic conditions.
Reduction: Sodium borohydride, lithium aluminum hydride; anhydrous conditions.
Substitution: Halides, alkoxides; polar aprotic solvents.
Major Products Formed: The major products formed from these reactions include oxidized derivatives, reduced forms, and substituted analogs of this compound, each with varying degrees of antimicrobial activity.
Scientific Research Applications
Antimicrobial Agent-22 has a wide range of scientific research applications, including:
Chemistry: Used as a model compound in studies of reaction mechanisms and kinetics.
Biology: Investigated for its effects on microbial cell structures and functions.
Medicine: Applied in the development of new antimicrobial therapies and treatments for resistant infections.
Industry: Utilized in the formulation of antimicrobial coatings, textiles, and packaging materials to prevent microbial contamination.
Mechanism of Action
The mechanism of action of Antimicrobial Agent-22 involves the disruption of microbial cell membranes and inhibition of essential enzymatic processes. The compound targets specific molecular pathways, such as protein synthesis and nucleic acid replication, leading to cell death. Its ability to bind to and deactivate key microbial enzymes makes it highly effective against a broad spectrum of pathogens.
Comparison with Similar Compounds
Antimicrobial Agent-21: Similar in structure but less effective against gram-negative bacteria.
Antimicrobial Agent-23: Exhibits higher potency but has a narrower spectrum of activity.
Antimicrobial Agent-24: Known for its stability but requires higher doses for effectiveness.
Uniqueness: Antimicrobial Agent-22 stands out due to its balanced spectrum of activity, high potency, and stability under various conditions. Its ability to target multiple microbial pathways simultaneously makes it a versatile and powerful antimicrobial agent.
Properties
Molecular Formula |
C15H16N4OS |
---|---|
Molecular Weight |
300.4 g/mol |
IUPAC Name |
1-butyl-3-(1,3-thiazol-2-yldiazenyl)indol-2-ol |
InChI |
InChI=1S/C15H16N4OS/c1-2-3-9-19-12-7-5-4-6-11(12)13(14(19)20)17-18-15-16-8-10-21-15/h4-8,10,20H,2-3,9H2,1H3 |
InChI Key |
PZDNXOPMAOCIEQ-UHFFFAOYSA-N |
Canonical SMILES |
CCCCN1C2=CC=CC=C2C(=C1O)N=NC3=NC=CS3 |
Origin of Product |
United States |
Disclaimer and Information on In-Vitro Research Products
Please be aware that all articles and product information presented on BenchChem are intended solely for informational purposes. The products available for purchase on BenchChem are specifically designed for in-vitro studies, which are conducted outside of living organisms. In-vitro studies, derived from the Latin term "in glass," involve experiments performed in controlled laboratory settings using cells or tissues. It is important to note that these products are not categorized as medicines or drugs, and they have not received approval from the FDA for the prevention, treatment, or cure of any medical condition, ailment, or disease. We must emphasize that any form of bodily introduction of these products into humans or animals is strictly prohibited by law. It is essential to adhere to these guidelines to ensure compliance with legal and ethical standards in research and experimentation.