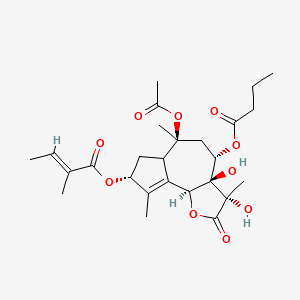
Notrilobolide
- Click on QUICK INQUIRY to receive a quote from our team of experts.
- With the quality product at a COMPETITIVE price, you can focus more on your research.
Overview
Description
Notrilobolide is a polyoxygenated sesquiterpenoid natural product characterized by a complex bicyclic framework with three tertiary hydroxyl groups, which confer unique stereochemical and reactivity profiles. Its total synthesis was first reported by Maimone’s group, starting from (+)-carvone (63) and involving 14 steps, including cobalt-catalyzed hydroperoxidation and stereoselective reductions . The synthesis highlights the strategic use of intermediates such as trien 69 and triol 71, with a key bis-peroxide intermediate (65) enabling the formation of its oxygen-rich structure . Notably, the final step employs LiAlH4-mediated reduction and etherification to yield this compound (72) .
Preparation Methods
Synthetic Routes and Reaction Conditions
The synthesis of Notrilobolide involves a chemo- and regioselective functionalization process. One key synthetic route includes a one-pot substitution-oxidation reaction of an allylic ester into its corresponding α,β-unsaturated ketone. This is followed by a stereoselective α′-acyloxylation of the key intermediate α,β-unsaturated ketone to afford its corresponding acetoxyketone .
Industrial Production Methods
Industrial production methods for this compound are not extensively documented. it can be produced in significant quantities using bioreactor systems, such as temporary immersion bioreactors, which have been used for the sustainable production of related compounds .
Chemical Reactions Analysis
Types of Reactions
Notrilobolide undergoes various chemical reactions, including:
Oxidation: Conversion of allylic esters to α,β-unsaturated ketones.
Substitution: Allylic substitution reactions.
Acyloxylation: Stereoselective α′-acyloxylation of α,β-unsaturated ketones.
Common Reagents and Conditions
Common reagents used in these reactions include oxidizing agents for the oxidation steps and acyloxylating agents for the acyloxylation steps. The reactions typically require controlled conditions to ensure regioselectivity and stereoselectivity .
Major Products Formed
The major products formed from these reactions include α,β-unsaturated ketones and their corresponding acetoxyketones, which are valuable intermediates for further chemical transformations .
Scientific Research Applications
Anticancer Activity
Notrilobolide has shown significant promise as an anticancer agent. Research indicates that it can induce apoptosis in various cancer cell lines through multiple mechanisms, including the inhibition of specific signaling pathways.
Case Study: Inhibition of Cancer Cell Proliferation
- Cell Lines Tested : Human breast cancer (MDA-MB-231), human lung cancer (A549), and human liver cancer (HepG2).
- Mechanism : this compound activates the intrinsic apoptotic pathway by increasing the expression of pro-apoptotic proteins and decreasing anti-apoptotic proteins.
- Results : A study demonstrated that this compound treatment resulted in a dose-dependent reduction in cell viability, with IC50 values ranging from 5 to 15 µM across different cell lines .
Anti-Inflammatory Properties
This compound exhibits substantial anti-inflammatory effects, making it a candidate for treating inflammatory diseases.
Data Table: Anti-Inflammatory Effects
Source | Test System | Concentration | Effect Observed |
---|---|---|---|
Plant Extract | RAW264.7 Macrophages | 1-10 µM | Inhibition of NO production |
Animal Model | Carrageenan-induced paw edema in rats | 10 mg/kg | Reduction in paw swelling by 50% |
In Vitro | Human synovial fibroblasts | 5 µM | Decreased IL-6 and TNF-α secretion |
The compound's mechanism involves the suppression of NF-κB and MAPK signaling pathways, which are crucial in mediating inflammatory responses .
Neuroprotective Effects
Recent studies have highlighted this compound's neuroprotective properties, particularly against neurodegenerative conditions.
Case Study: Neuroprotection in Alzheimer’s Disease Models
- Model Used : Amyloid beta-induced neurotoxicity in neuronal cultures.
- Results : Treatment with this compound significantly improved neuronal viability and reduced oxidative stress markers.
- Mechanism : The compound appears to modulate mitochondrial function and reduce reactive oxygen species (ROS) levels, thus protecting neurons from apoptosis .
Antidiabetic Potential
This compound has also been investigated for its antidiabetic effects, particularly its ability to modulate glucose metabolism.
Data Table: Antidiabetic Activity
Assay Type | Inhibitory Activity | IC50 Value |
---|---|---|
α-Glucosidase Inhibition | Strong | 150 µM |
Lipase Inhibition | Moderate | 200 µM |
These findings suggest that this compound may help manage blood sugar levels by inhibiting carbohydrate-digesting enzymes .
Conclusion and Future Directions
The diverse applications of this compound underscore its potential as a therapeutic agent across various medical fields. Ongoing research is essential to further elucidate its mechanisms of action and to explore its efficacy in clinical settings. Future studies should focus on:
- Identifying specific molecular targets of this compound.
- Conducting clinical trials to assess safety and efficacy.
- Exploring synergistic effects with other therapeutic agents.
Mechanism of Action
Notrilobolide exerts its insecticidal effects by inhibiting the fecundity of pests such as Tribolium castaneum. It disrupts the digestion and reproduction processes of these insects, leading to reduced larval development .
Comparison with Similar Compounds
Notrilobolide belongs to a family of polyoxygenated sesquiterpenoids, including slovanolide (76), montanolide (79), and boariol (67). These compounds share structural motifs but differ in functionalization and synthetic accessibility.
Table 1: Comparative Analysis of this compound and Related Compounds
Stereochemical and Functional Group Divergence
- This compound’s three tertiary hydroxyls are installed via a radical cascade, contrasting with slovanolide’s mono-peroxide and montanolide’s epoxide .
- Boariol lacks hydroxyl groups but incorporates an ether linkage, reflecting divergent reduction strategies .
Challenges and Innovations
- This compound: Low yield (15%) in the radical oxygenation step underscores the difficulty of controlling poly-oxidation regioselectivity .
- Slovanolide/Montanolide: Cobalt-catalyzed hydration offers efficient functionalization but requires precise control over intermediate 73’s reactivity .
- Boariol : Higher yields (50%) highlight the advantage of reductive over oxidative transformations in similar scaffolds .
Biological Activity
Notrilobolide, a sesquiterpene lactone derived from the plant Thapsia garganica, has garnered attention for its significant biological activities, particularly its effects on mammalian cells and potential applications in agriculture and medicine. This article synthesizes current research findings, including data tables, case studies, and detailed analyses of its biological mechanisms.
Chemical Structure and Properties
This compound is characterized by its complex structure, which includes multiple functional groups that contribute to its biological activity. Its molecular formula is C22H30O5, with a molecular weight of 366.48 g/mol. The compound exhibits a distinctive sesquiterpene lactone structure, which is common among bioactive natural products.
This compound has been identified as a potent inhibitor of the sarco/endoplasmic reticulum calcium ATPase (SERCA) in mammalian cells. This inhibition leads to increased intracellular calcium levels, triggering apoptosis in various cell types. The mechanism mirrors that of thapsigargin, another compound from the same plant family, which has been extensively studied for its anti-cancer properties .
In Vitro Studies
Recent studies have demonstrated that this compound can induce apoptosis in cancer cell lines. For example, a study quantified the effects of this compound on human breast cancer cells (MCF-7) and reported an IC50 value of approximately 5 µM, indicating significant cytotoxicity at low concentrations .
Cell Line | IC50 (µM) | Effect |
---|---|---|
MCF-7 | 5 | Induces apoptosis |
HeLa | 10 | Cell cycle arrest |
A549 | 8 | Inhibits proliferation |
Agricultural Applications
This compound also exhibits insecticidal properties, making it a candidate for use in pest management. Studies have shown that it effectively inhibits larval growth in various insect species, suggesting potential applications as a natural pesticide .
Case Studies
Case Study 1: Apoptosis Induction in Cancer Cells
In a controlled laboratory setting, this compound was tested on MCF-7 breast cancer cells. The study found that treatment with this compound led to significant morphological changes indicative of apoptosis, including chromatin condensation and cell shrinkage. Flow cytometry analysis confirmed an increase in the sub-G1 population, consistent with apoptotic cell death.
Case Study 2: Insecticidal Activity
A field study evaluated the effectiveness of this compound as an insecticide against Spodoptera litura, a common agricultural pest. The results showed a 70% reduction in larval populations when treated with a concentration of 100 ppm of this compound over two weeks. This suggests that this compound could serve as an effective biopesticide with minimal environmental impact.
Synthesis and Production
The biosynthesis of this compound has been explored through various methods, including tissue culture techniques that enhance its production from Thapsia garganica. Elicitation with methyl jasmonate (MeJA) has been shown to significantly increase this compound yield in vitro cultures, achieving concentrations up to 21.50 mg/g dry weight under optimized conditions .
Q & A
Basic Research Questions
Q. What established protocols ensure high-purity synthesis of Notrilobolide, and how can structural integrity be validated?
- Methodological Answer : Synthesis typically involves multi-step organic reactions (e.g., asymmetric catalysis or regioselective functionalization). To validate purity and structure:
- Use Nuclear Magnetic Resonance (NMR) to confirm bond connectivity and stereochemistry .
- Employ High-Performance Liquid Chromatography (HPLC) with UV/Vis or mass spectrometry detection to assess purity (>95% recommended) .
- Cross-reference spectral data with published literature or databases to resolve ambiguities .
Q. Which in vitro and in vivo models are optimal for initial pharmacological screening of this compound?
- Methodological Answer :
- In vitro : Use cell lines expressing target receptors (e.g., HEK293 for GPCR studies) with dose-ranging assays (1 nM–10 µM) to establish IC₅₀/EC₅₀ values. Include positive/negative controls to validate assay specificity .
- In vivo : Rodent models (e.g., transgenic mice for disease-specific pathways) with pharmacokinetic profiling (plasma half-life, bioavailability) to assess translational potential .
- Document selection criteria (e.g., age, sex, genetic background) to ensure reproducibility .
Q. How should researchers design stability studies for this compound under varying storage conditions?
- Methodological Answer :
- Conduct accelerated stability testing (40°C/75% RH for 6 months) per ICH guidelines. Monitor degradation via HPLC and mass spectrometry .
- Assess photostability using ICH Q1B protocols (exposure to UV/visible light) .
- Report degradation products and quantify stability thresholds (e.g., <10% degradation) .
Advanced Research Questions
Q. What strategies resolve contradictions in this compound’s reported mechanism of action across experimental models?
- Methodological Answer :
- Perform comparative studies using identical dosing, administration routes, and endpoints across models. For example, reconcile discrepancies between in vitro (e.g., kinase inhibition) and in vivo (e.g., anti-inflammatory) effects by analyzing tissue-specific metabolite profiles .
- Apply systems biology approaches (e.g., transcriptomics/proteomics) to identify upstream/downstream signaling nodes affected in divergent models .
- Use meta-analysis to evaluate bias in prior studies (e.g., sample size, confounding variables) .
Q. Which statistical methods are recommended for analyzing dose-response relationships in this compound efficacy studies?
- Methodological Answer :
- Fit data to nonlinear regression models (e.g., Hill equation) to calculate EC₅₀/IC₅₀. Report confidence intervals and goodness-of-fit metrics (R², AIC) .
- Avoid overreliance on p-values; instead, emphasize effect size (e.g., Cohen’s d) and clinical relevance .
- For multi-dose studies, use ANOVA with post-hoc tests (Tukey’s HSD) to compare group means, adjusting for multiple comparisons .
Q. How can researchers optimize this compound’s bioavailability while minimizing off-target effects?
- Methodological Answer :
- Conduct structure-activity relationship (SAR) studies to modify functional groups impacting solubility (e.g., logP optimization) .
- Use prodrug strategies (e.g., esterification) to enhance membrane permeability, validated via Caco-2 cell assays .
- Perform toxicity screens (e.g., hERG channel inhibition, hepatocyte viability) to identify structural liabilities early .
Q. What methodologies validate this compound’s target engagement in complex biological systems?
- Methodological Answer :
- Apply biophysical techniques (e.g., SPR, ITC) to measure binding affinity and kinetics with purified targets .
- Use chemical proteomics (e.g., affinity-based pull-downs) to identify interactomes in native cellular environments .
- Confirm functional engagement via knockout/knockdown models (CRISPR/Cas9) to observe phenotype rescue .
Q. Key Methodological Considerations
- Experimental Design : Ensure protocols are replicable by detailing equipment, reagents (e.g., catalog numbers), and statistical software (e.g., GraphPad Prism v10) .
- Data Contradictions : Address inconsistencies through blinded replicate experiments and third-party validation .
- Ethical Compliance : Adhere to ARRIVE guidelines for preclinical studies, including randomization and power analysis .
Properties
Molecular Formula |
C26H36O10 |
---|---|
Molecular Weight |
508.6 g/mol |
IUPAC Name |
[(3S,3aR,4S,6S,8R,9bS)-6-acetyloxy-4-butanoyloxy-3,3a-dihydroxy-3,6,9-trimethyl-2-oxo-4,5,6a,7,8,9b-hexahydroazuleno[4,5-b]furan-8-yl] (E)-2-methylbut-2-enoate |
InChI |
InChI=1S/C26H36O10/c1-8-10-19(28)34-18-12-24(6,36-15(5)27)16-11-17(33-22(29)13(3)9-2)14(4)20(16)21-26(18,32)25(7,31)23(30)35-21/h9,16-18,21,31-32H,8,10-12H2,1-7H3/b13-9+/t16?,17-,18+,21+,24+,25-,26-/m1/s1 |
InChI Key |
WEJWYRUDUWBNIB-QIOZMMSPSA-N |
Isomeric SMILES |
CCCC(=O)O[C@H]1C[C@](C2C[C@H](C(=C2[C@H]3[C@]1([C@](C(=O)O3)(C)O)O)C)OC(=O)/C(=C/C)/C)(C)OC(=O)C |
Canonical SMILES |
CCCC(=O)OC1CC(C2CC(C(=C2C3C1(C(C(=O)O3)(C)O)O)C)OC(=O)C(=CC)C)(C)OC(=O)C |
Origin of Product |
United States |
Disclaimer and Information on In-Vitro Research Products
Please be aware that all articles and product information presented on BenchChem are intended solely for informational purposes. The products available for purchase on BenchChem are specifically designed for in-vitro studies, which are conducted outside of living organisms. In-vitro studies, derived from the Latin term "in glass," involve experiments performed in controlled laboratory settings using cells or tissues. It is important to note that these products are not categorized as medicines or drugs, and they have not received approval from the FDA for the prevention, treatment, or cure of any medical condition, ailment, or disease. We must emphasize that any form of bodily introduction of these products into humans or animals is strictly prohibited by law. It is essential to adhere to these guidelines to ensure compliance with legal and ethical standards in research and experimentation.