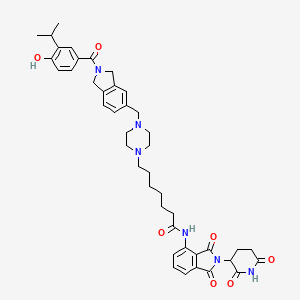
PROTAC Hsp90|A degrader 1
- Click on QUICK INQUIRY to receive a quote from our team of experts.
- With the quality product at a COMPETITIVE price, you can focus more on your research.
Overview
Description
Preparation Methods
Synthetic Routes and Reaction Conditions
The synthesis of PROTAC Hsp90|A degrader 1 involves the design and evaluation of proteolysis-targeting chimeras (PROTACs). The synthetic route typically includes the conjugation of a ligand for the target protein (Hsp90α) with a ligand for an E3 ubiquitin ligase, connected by a linker. The reaction conditions often involve the use of organic solvents and catalysts to facilitate the formation of the desired product .
Industrial Production Methods
Industrial production methods for this compound are not extensively documented. the general approach would involve scaling up the laboratory synthesis process, optimizing reaction conditions for higher yields, and ensuring the purity of the final product through various purification techniques such as chromatography .
Chemical Reactions Analysis
Types of Reactions
PROTAC Hsp90|A degrader 1 undergoes several types of chemical reactions, including:
Oxidation: Involves the addition of oxygen or the removal of hydrogen.
Reduction: Involves the addition of hydrogen or the removal of oxygen.
Substitution: Involves the replacement of one functional group with another.
Common Reagents and Conditions
Common reagents used in these reactions include oxidizing agents like hydrogen peroxide, reducing agents like sodium borohydride, and various organic solvents. The reaction conditions typically involve controlled temperatures and pH levels to ensure the desired chemical transformations .
Major Products Formed
The major products formed from these reactions depend on the specific conditions and reagents used. For instance, oxidation reactions may yield oxidized derivatives of the compound, while reduction reactions may produce reduced forms of the compound .
Scientific Research Applications
PROTAC Hsp90|A degrader 1 has a wide range of scientific research applications, including:
Chemistry: Used as a model compound to study the mechanisms of protein degradation and the design of PROTACs.
Biology: Employed in cellular studies to investigate the role of Hsp90α in various biological processes.
Medicine: Explored as a potential therapeutic agent for the treatment of breast cancer and other cancers by targeting Hsp90α for degradation.
Industry: Utilized in the development of new drugs and therapeutic strategies based on targeted protein degradation
Mechanism of Action
The mechanism of action of PROTAC Hsp90|A degrader 1 involves the recruitment of an E3 ubiquitin ligase to the target protein Hsp90α. This leads to the ubiquitination of Hsp90α, marking it for degradation by the proteasome. The degradation of Hsp90α disrupts its function in stabilizing and activating client proteins, thereby inhibiting the proliferation of cancer cells .
Comparison with Similar Compounds
Similar Compounds
VER49009: An effective Hsp90 inhibitor with an IC50 of 25 nM.
HSF1A: A cell-permeable activator of HSF1 that protects mammalian cells against stress-induced apoptosis.
Uniqueness
PROTAC Hsp90|A degrader 1 is unique in its ability to selectively degrade Hsp90α through the PROTAC mechanism, which involves the recruitment of an E3 ubiquitin ligase and subsequent proteasomal degradation. This approach offers advantages over traditional inhibitors by completely removing the target protein rather than merely inhibiting its activity .
Properties
Molecular Formula |
C43H50N6O7 |
---|---|
Molecular Weight |
762.9 g/mol |
IUPAC Name |
N-[2-(2,6-dioxopiperidin-3-yl)-1,3-dioxoisoindol-4-yl]-7-[4-[[2-(4-hydroxy-3-propan-2-ylbenzoyl)-1,3-dihydroisoindol-5-yl]methyl]piperazin-1-yl]heptanamide |
InChI |
InChI=1S/C43H50N6O7/c1-27(2)33-23-29(13-15-36(33)50)41(54)48-25-30-12-11-28(22-31(30)26-48)24-47-20-18-46(19-21-47)17-6-4-3-5-10-37(51)44-34-9-7-8-32-39(34)43(56)49(42(32)55)35-14-16-38(52)45-40(35)53/h7-9,11-13,15,22-23,27,35,50H,3-6,10,14,16-21,24-26H2,1-2H3,(H,44,51)(H,45,52,53) |
InChI Key |
LHEOCGZFVGFDNZ-UHFFFAOYSA-N |
Canonical SMILES |
CC(C)C1=C(C=CC(=C1)C(=O)N2CC3=C(C2)C=C(C=C3)CN4CCN(CC4)CCCCCCC(=O)NC5=CC=CC6=C5C(=O)N(C6=O)C7CCC(=O)NC7=O)O |
Origin of Product |
United States |
Disclaimer and Information on In-Vitro Research Products
Please be aware that all articles and product information presented on BenchChem are intended solely for informational purposes. The products available for purchase on BenchChem are specifically designed for in-vitro studies, which are conducted outside of living organisms. In-vitro studies, derived from the Latin term "in glass," involve experiments performed in controlled laboratory settings using cells or tissues. It is important to note that these products are not categorized as medicines or drugs, and they have not received approval from the FDA for the prevention, treatment, or cure of any medical condition, ailment, or disease. We must emphasize that any form of bodily introduction of these products into humans or animals is strictly prohibited by law. It is essential to adhere to these guidelines to ensure compliance with legal and ethical standards in research and experimentation.