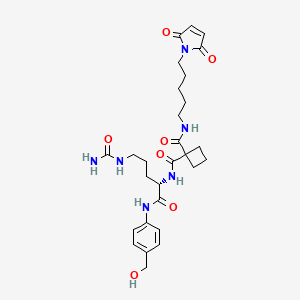
cBu-Cit-OH
- Click on QUICK INQUIRY to receive a quote from our team of experts.
- With the quality product at a COMPETITIVE price, you can focus more on your research.
Overview
Description
Preparation Methods
Synthetic Routes and Reaction Conditions: The synthesis of cBu-Cit-OH involves the formation of a cyclobutane ring and the subsequent attachment of citrulline and para-aminobenzoic acid (PAB). The reaction conditions typically include the use of organic solvents and catalysts to facilitate the formation of the cyclobutane ring and the subsequent functionalization steps .
Industrial Production Methods: Industrial production of this compound follows similar synthetic routes but on a larger scale. The process involves stringent quality control measures to ensure the purity and consistency of the final product. The use of automated reactors and continuous flow systems can enhance the efficiency and scalability of the production process .
Chemical Reactions Analysis
Types of Reactions: cBu-Cit-OH primarily undergoes cleavage reactions, particularly in the presence of proteases such as cathepsin B. This cleavage releases the cytotoxic payload from the ADC, enabling targeted drug delivery .
Common Reagents and Conditions: The cleavage reactions typically occur under physiological conditions, with the presence of specific proteases being the key factor. The reaction conditions are optimized to ensure selective cleavage in the tumor microenvironment .
Major Products Formed: The major product formed from the cleavage of this compound is the active cytotoxic drug, which is released at the target site to exert its therapeutic effects .
Scientific Research Applications
cBu-Cit-OH is extensively used in the development of antibody-drug conjugates for targeted cancer therapy. Its role as a linker molecule is crucial in ensuring the stability of the ADC in the bloodstream and the selective release of the cytotoxic payload in the tumor microenvironment . Additionally, this compound has applications in the study of protease-specific cleavage mechanisms and the development of novel linker technologies for improved drug delivery systems .
Mechanism of Action
The mechanism of action of cBu-Cit-OH involves its cleavage by proteases such as cathepsin B. This cleavage releases the cytotoxic drug from the ADC, allowing it to exert its effects on the cancer cells. The molecular targets and pathways involved include the binding of the ADC to specific antigens on the cancer cell surface, followed by internalization and cleavage of the linker to release the drug .
Comparison with Similar Compounds
- Valine-citrulline (Val-Cit) linker
- Glycine-glycine-phenylalanine-glycine (Gly-Gly-Phe-Gly) linker
- Disulfide linkers
Comparison: Compared to other linkers such as Valine-citrulline and Glycine-glycine-phenylalanine-glycine, cBu-Cit-OH exhibits enhanced protease specificity, particularly for cathepsin B. This specificity results in more efficient and selective drug release in the tumor microenvironment . Additionally, this compound demonstrates greater stability in the bloodstream, reducing off-target toxicity and improving the therapeutic window of the ADC .
Properties
Molecular Formula |
C28H38N6O7 |
---|---|
Molecular Weight |
570.6 g/mol |
IUPAC Name |
1-N'-[(2S)-5-(carbamoylamino)-1-[4-(hydroxymethyl)anilino]-1-oxopentan-2-yl]-1-N-[5-(2,5-dioxopyrrol-1-yl)pentyl]cyclobutane-1,1-dicarboxamide |
InChI |
InChI=1S/C28H38N6O7/c29-27(41)31-16-4-6-21(24(38)32-20-9-7-19(18-35)8-10-20)33-26(40)28(13-5-14-28)25(39)30-15-2-1-3-17-34-22(36)11-12-23(34)37/h7-12,21,35H,1-6,13-18H2,(H,30,39)(H,32,38)(H,33,40)(H3,29,31,41)/t21-/m0/s1 |
InChI Key |
HLAKJCQSHGCDPL-NRFANRHFSA-N |
Isomeric SMILES |
C1CC(C1)(C(=O)NCCCCCN2C(=O)C=CC2=O)C(=O)N[C@@H](CCCNC(=O)N)C(=O)NC3=CC=C(C=C3)CO |
Canonical SMILES |
C1CC(C1)(C(=O)NCCCCCN2C(=O)C=CC2=O)C(=O)NC(CCCNC(=O)N)C(=O)NC3=CC=C(C=C3)CO |
Origin of Product |
United States |
Disclaimer and Information on In-Vitro Research Products
Please be aware that all articles and product information presented on BenchChem are intended solely for informational purposes. The products available for purchase on BenchChem are specifically designed for in-vitro studies, which are conducted outside of living organisms. In-vitro studies, derived from the Latin term "in glass," involve experiments performed in controlled laboratory settings using cells or tissues. It is important to note that these products are not categorized as medicines or drugs, and they have not received approval from the FDA for the prevention, treatment, or cure of any medical condition, ailment, or disease. We must emphasize that any form of bodily introduction of these products into humans or animals is strictly prohibited by law. It is essential to adhere to these guidelines to ensure compliance with legal and ethical standards in research and experimentation.