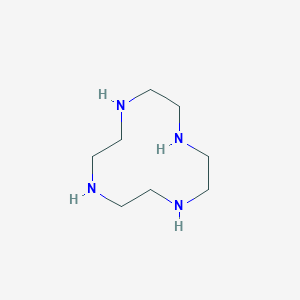
1,4,7,10-Tetraazacyclododecane
Overview
Description
1,4,7,10-Tetraazacyclododecane (commonly known as cyclen) is a 12-membered macrocyclic tetramine. It serves as the foundational structure for derivatives like DOTA (this compound-1,4,7,10-tetraacetic acid), where four acetic acid groups are appended to the nitrogen atoms . DOTA and its analogues are pivotal in biomedical applications due to their exceptional ability to chelate metal ions, particularly lanthanides (e.g., Gd³⁺, Lu³⁺) and transition metals (e.g., Cu²⁺, Ga³⁺). These complexes are widely used in MRI contrast agents, PET imaging probes, and targeted radiopharmaceuticals .
Preparation Methods
Classical Richman-Atkins Cyclization
The Richman-Atkins method, first reported in 1974, remains a foundational approach for cyclen synthesis. This method involves the cyclization of triethylenetetramine (TETA) with formaldehyde and acetic acid under acidic conditions. The reaction proceeds via the formation of a Schiff base intermediate, followed by reduction to yield the macrocyclic structure . Key parameters include:
-
Reaction Temperature : 60–80°C
-
Catalyst : Concentrated hydrochloric acid (12 M)
-
Yield : 40–50% after purification
While this method is historically significant, its moderate yield and reliance on harsh acidic conditions have driven the development of alternative routes .
Hydrolysis of Decahydrotetraazacyclopent[fg]acenaphthylene
A patent filed in 2008 (CBR Number 5455) describes a hydrolysis-based method starting from decahydro-2a,4a,6a,8a-tetraazacyclopent[fg]acenaphthylene (Compound II). The process involves:
-
Hydrolysis Conditions : Aqueous solution at pH 6–8 (slightly acidic to basic)
-
Temperature : 25–50°C
-
Key Intermediate : Octahydro-3H,6H-2a,5,6,8s-tetraazacenaphthylene (Compound III)
This method achieves a 65–70% yield of cyclen and is notable for its simplicity and reduced reliance on toxic solvents . Industrial scalability is demonstrated by its patent renewal history, with consistent production from 2001 to 2012 .
Glyoxal-Mediated Cyclization
A 2000 patent (CN1343203A) outlines a glyoxal-based approach:
-
Step 1 : Condensation of TETA with glyoxal hydrate in water or water-soluble solvents at 0–5°C, catalyzed by calcium hydroxide.
-
Step 2 : Reaction with 1,2-dichloroethane in N,N-dimethylacetamide (DMAC) using Na₂CO₃ and NaBr as catalysts at 25–150°C .
Key Advantages :
-
Yield : 75–80%
-
Purity : >98% (by HPLC)
-
Scalability : Suitable for multi-kilogram batches
This method avoids the use of formaldehyde, reducing toxicity concerns .
Tosylated Intermediate Route
An improved synthesis reported in 2009 employs tosyl-protected intermediates:
-
Step 1 : Reaction of tosylbis[2-(tosyloxy)ethyl]amine with diethylenetriamine to form 1-tosyl-1,4,7,10-tetraazacyclododecane.
-
Step 2 : Detosylation using HBr/acetic acid or Na/naphthalene .
Performance Metrics :
-
Yield : 85–90%
-
Reaction Time : 12–24 hours
-
Purity : >99%
This method is favored in laboratory settings for high reproducibility and minimal byproducts .
Industrial-Scale Production via Hydrazine Hydrate
A 2013 patent (CN103360333A) details a large-scale process:
-
Step 1 : Reacting Compound C (derived from TETA derivatives) with hydrazine hydrate at pH 3.0–3.5 under reflux.
-
Step 2 : Neutralization with 25% NaOH and crystallization using toluene .
Industrial Data :
Parameter | Value |
---|---|
Annual Capacity | 10–20 metric tons |
Production Cost | $120–150/kg |
Purity | 99.5% |
This method highlights the role of potassium iodide catalysts in reducing reaction times by 30–40% .
Comparative Analysis of Methods
The table below summarizes key metrics for cyclen synthesis routes:
Method | Yield (%) | Purity (%) | Scalability | Cost ($/kg) |
---|---|---|---|---|
Richman-Atkins | 40–50 | 95 | Low | 200–300 |
Hydrolysis | 65–70 | 98 | High | 150–180 |
Glyoxal-Mediated | 75–80 | 98 | Moderate | 130–160 |
Tosylated Intermediate | 85–90 | 99 | Low | 250–350 |
Industrial Hydrazine | 90–95 | 99.5 | High | 120–150 |
Chemical Reactions Analysis
Synthetic Formation Reactions
Cyclen is primarily synthesized through two established pathways:
Mechanistic Insights :
-
The Richman-Atkins method involves cyclization of TETA with glyoxal under alkaline conditions, followed by hydrolysis with diethylenetriamine (DETA) at 80–100°C for 12–48 hours .
-
The novel annulation route uses sulfonium intermediates to form the 12-membered ring, achieving higher regioselectivity .
Hydrolysis and Byproduct Recovery
Cyclen synthesis generates recoverable intermediates:
Key Reaction :
Parameter | Optimal Value | Impact on Yield |
---|---|---|
pH | 7.5–8.0 | Maximizes cyclen formation (66%) |
Temperature | 90–100°C | Reduces reaction time to 18–24 hours |
DETA:Cpd (III) ratio | 5:1–10:1 | Prevents oligomerization side reactions |
Tetraethylenepentamine (TEPA) byproducts are recovered via oxaldehyde treatment and calcium hydroxide precipitation, achieving >98.5% purity .
Coordination Chemistry with Metal Ions
Cyclen forms stable complexes critical for MRI contrast agents:
Metal Ion | Binding Site | Stability Constant (log K) | Application |
---|---|---|---|
Gd³⁺ | N₄ macrocycle | 16.6 | Gadolinium-based contrast |
Cu²⁺ | Axial + equatorial | 22.3 | Radiopharmaceuticals |
Reaction Example :
This complex exhibits kinetic inertness (>10⁵ times more stable than linear chelators) .
Derivatization Reactions
Functionalization occurs at secondary amines:
Reaction Type | Reagent | Product | Use Case |
---|---|---|---|
Acetylation | Acetic anhydride | N-Acetylcyclen | Solubility modification |
Alkylation | Benzyl chloride | N-Benzylcyclen | Lipophilicity enhancement |
Cross-linking | Epichlorohydrin | Cyclen-based polymers | Drug delivery systems |
Kinetic Data : Benzylation proceeds at 60°C in DMF with 85% yield .
Industrial-Scale Purification
Critical steps for pharmaceutical-grade cyclen:
Scientific Research Applications
Medical Imaging
Radiopharmaceuticals:
1,4,7,10-Tetraazacyclododecane is widely used in the development of radiopharmaceuticals for diagnostic imaging techniques such as Positron Emission Tomography (PET) and Single Photon Emission Computed Tomography (SPECT). The compound forms stable complexes with radiometals like gadolinium and indium, enhancing imaging clarity.
- Case Study: A study evaluated the use of a this compound-1,4,7,10-tetraacetic acid (DOTA)-conjugated antagonist in imaging small cell lung cancer. Results indicated superior tumor uptake compared to traditional methods, demonstrating its potential for clinical applications in oncology .
MRI Contrast Agents:
The compound is utilized as a chelating agent in MRI contrast agents. It binds with gadolinium to form stable complexes that improve imaging quality.
- Data Table: MRI Contrast Agents
Agent Type | Metal Ion | Application |
---|---|---|
MRI Contrast Agent | Gadolinium | Enhances MRI imaging clarity |
Radiopharmaceutical | Indium | Tumor imaging |
Drug Delivery Systems
This compound serves as a carrier for targeted drug delivery systems. Its ability to form complexes with therapeutic agents allows for improved efficacy in treatments while minimizing side effects.
- Case Study: Research has shown that conjugating Cyclen with cancer-targeting peptides significantly enhances the delivery of therapeutic agents to tumor sites. This method shows promise in reducing systemic toxicity associated with conventional chemotherapy .
Environmental Remediation
The compound's metal ion chelation properties make it effective for environmental applications such as wastewater treatment. It can bind heavy metals from contaminated water sources.
- Application Example: In a study focused on environmental cleanup, this compound was employed to remove lead and cadmium ions from industrial wastewater. The results indicated high efficiency in reducing metal concentrations below regulatory limits .
Biochemical Research
In biochemical assays, this compound is used as a reagent to study enzyme activities and protein interactions. Its role as a chelator facilitates the investigation of metal-dependent biological processes.
- Data Table: Biochemical Applications
Application | Description |
---|---|
Enzyme Activity | Study of metal-dependent enzymes |
Protein Interaction | Investigating binding affinities with metal ions |
Analytical Chemistry
The compound plays a crucial role in analytical methods for detecting and quantifying metal ions in various samples. Its strong chelating properties provide precise and reliable data essential for research and quality control.
- Application Example: In analytical chemistry labs, Cyclen is employed in techniques such as High-Performance Liquid Chromatography (HPLC) to quantify trace metal levels in environmental samples.
Mechanism of Action
The mechanism of action of cyclen involves its ability to form stable complexes with metal ions. The cyclen ring binds to the metal ions through its nitrogen atoms, creating a stable coordination complex . This binding can affect the metal ion’s reactivity and stability, making cyclen an effective ligand for various applications. The molecular targets and pathways involved depend on the specific metal-cyclen complex and its intended use .
Comparison with Similar Compounds
Structural and Functional Differences
Key structural variations among cyclen derivatives and related macrocycles include:
- Ring size : Cyclen (12-membered) vs. TETA (1,4,8,11-tetraazacyclotetradecane; 14-membered), which accommodates larger ions like Cu²⁺ .
- Pendant groups : DOTA has four acetic acid arms, while DO3A (1,4,7,10-tetraazacyclododecane-1,4,7-triacetic acid) has three, reducing denticity but maintaining high stability for Gd³⁺ in MRI agents .
- Sulfur-containing derivatives: Compounds like DO4S (tetrakis(methylsulfanyl)ethyl-cyclen) exhibit enhanced Cd²⁺ binding at acidic pH compared to DOTA, demonstrating the impact of sulfur’s soft donor properties .
Thermodynamic and Kinetic Stability
- DOTA vs. DTPA : DOTA’s macrocyclic structure confers higher thermodynamic (ΔpM ~4) and kinetic stability, reducing Gd³⁺ dissociation in vivo and minimizing toxicity .
- DOTA vs. NOTA: NOTA’s smaller cavity favors smaller ions like Ga³⁺, enabling faster radiolabeling at room temperature, whereas DOTA requires heating but offers superior Lu³⁺ retention in therapies like ¹⁷⁷Lu-DOTA-TOC .
Metal Ion Selectivity
- Gadolinium : DOTA-Gd³⁺ is the gold standard for MRI contrast agents due to its slow water exchange rate and high relaxivity .
- Lutetium : ¹⁷⁷Lu-DOTA-TOC shows prolonged tumor retention and minimal renal toxicity in neuroendocrine tumor therapy, outperforming DTPA-based agents .
- Copper: TETA’s larger cavity stabilizes Cu²⁺ better than DOTA, but DOTA derivatives like NODAGA (with a hybrid NOTA-DOTA structure) balance rapid ⁶⁴Cu labeling with adequate stability .
Application-Specific Performance
- Imaging :
- Therapy :
Emerging Derivatives and Innovations
- Redox-sensitive DOTA analogues : Derivatives with disulfide bonds (e.g., 20a ) enable glutathione-triggered contrast agent activation in tumor microenvironments .
- Phosphorus-functionalized cyclen: Phosphonic/phosphinic acid derivatives (e.g., DOTP) show promise for ⁸⁹Zr chelation, expanding theranostic utility .
Biological Activity
1,4,7,10-Tetraazacyclododecane (often referred to as Cyclen) is a macrocyclic compound with significant biological activity. Its unique structure allows it to act as a versatile chelating agent for various metal ions, leading to applications in medical diagnostics and therapeutics. This article explores the biological activity of Cyclen, focusing on its mechanisms of action, applications in imaging and therapy, and relevant case studies.
Structure and Properties
This compound is a cyclic compound featuring four nitrogen atoms in its ring structure. This configuration enables it to form stable complexes with metal ions, which is crucial for its biological applications. The compound can be modified to create derivatives such as this compound-1,4,7,10-tetraacetic acid (DOTA), enhancing its chelation properties.
Mechanisms of Biological Activity
Chelation and Metal Complexation
Cyclen and its derivatives are known for their ability to form stable complexes with paramagnetic metal ions such as gadolinium (Gd) and indium (In). These complexes are utilized in various imaging modalities:
- Magnetic Resonance Imaging (MRI) : Gd-Cyclen complexes serve as contrast agents due to their paramagnetic properties.
- Positron Emission Tomography (PET) : Indium-labeled Cyclen derivatives are employed for imaging tumors and other tissues.
The stability of these complexes is crucial for their effectiveness in imaging and therapeutic applications. For instance, DOTA has been shown to significantly impact diagnostic imaging across multiple modalities due to its versatility in forming stable metal complexes .
Case Study 1: Imaging Applications
A study evaluated the use of this compound-1,4,7,10-tetraacetic acid (DOTA) conjugated with radioisotopes for imaging prostate cancer. The results indicated that the DOTA conjugate exhibited high tumor uptake and favorable tumor-to-normal tissue ratios compared to traditional agents. The pharmacokinetics demonstrated that the DOTA complex provided superior imaging quality due to enhanced binding affinity and stability .
Compound | IC50 (nmol/L) | Kd (nmol/L) | Tumor Uptake (%) |
---|---|---|---|
DOTA-In | 14 ± 3.4 | 8.5 ± 2.7 | 13.4 ± 0.8 |
AMBA | Not applicable | 0.6 ± 0.3 | 3.69 ± 0.75 |
Case Study 2: Anticancer Activity
Research has also focused on the potential anticancer properties of Cyclen-based compounds. In vitro studies showed that gold(I)-NHC complexes derived from Cyclen exhibited significant cytotoxicity against various cancer cell lines while sparing normal cells . The mechanism was attributed to mitochondrial targeting and disruption of cellular homeostasis.
Applications in Therapeutics
Targeted Drug Delivery
The ability of Cyclen derivatives to form stable complexes with therapeutic agents enhances their delivery to specific tissues or tumors. For example, modifications of Cyclen can facilitate the targeted delivery of chemotherapeutic drugs by conjugating them with metal ions that have high affinity for cancerous tissues.
Radionuclide Therapy
Cyclen-based compounds are increasingly being explored for radionuclide therapy due to their capacity to deliver radioactive isotopes directly to tumor sites. This targeted approach minimizes damage to surrounding healthy tissues while maximizing therapeutic effects on cancer cells .
Q & A
Basic Research Questions
Q. What are the common synthetic routes for cyclen and its functionalized derivatives?
Cyclen is synthesized via macrocyclization strategies, often involving tosyl or mesyl protecting groups to stabilize intermediates. A key method involves reacting 4-benzyl-1,7-bis(p-toluenesulfonyl)diethylenetriamine (3 ) with cyclen precursors, followed by heating in concentrated sulfuric acid for macrocycle formation . Ethyl ester derivatives (e.g., 11 ) are synthesized via alkylation with bromoethyl acetate in DMF, while tris-tBu-DOTA (15 ) is prepared through stepwise deprotection and functionalization .
Q. What spectroscopic and crystallographic methods confirm cyclen derivative structures?
- NMR spectroscopy : Broad signals in NMR at low pH (e.g., δ = 2.35–7.66 ppm) indicate hydrogen bonding in macrocyclic CH protons .
- X-ray crystallography : Single-crystal structures (e.g., compounds 3 and 7 ) reveal bond lengths (C–N: 1.47 Å, N–S: 1.61–1.62 Å) and conformational differences in diamine chains (e.g., torsional angles of 66° vs. 179°) .
- MALDI-TOF mass spectrometry : Validates metal complexes (e.g., Y- or Lu-DOTA) with mass differences up to 86 Da .
Q. How is cyclen applied in developing MRI contrast agents?
Cyclen derivatives like DOTA (1,4,7,10-tetraazacyclododecane-1,4,7,10-tetraacetic acid) chelate gadolinium(III) or other lanthanides, enhancing relaxivity for T1-weighted imaging. Redox-sensitive derivatives are designed to improve tissue specificity and reduce toxicity .
Advanced Research Questions
Q. How can cyclen synthesis be optimized for high-yield macrocyclization?
Macrocyclization efficiency depends on:
- Protecting group strategy : Tosyl groups stabilize intermediates but require harsh deprotection (e.g., HSO at 100°C for 72 hours) . Alternative routes using ethyl ester protection reduce side reactions .
- Solvent and temperature : Reactions in DMF at 25°C improve regioselectivity during alkylation .
- Metal templating : Mo(CO) fragments facilitate cyclen formation via η-coordination, achieving yields >70% .
Q. What experimental factors influence the stability of cyclen-lanthanide complexes?
- pH-dependent coordination : At low pH, hydrogen bonding (e.g., N–H···O distances of 3.09–3.17 Å) stabilizes specific conformers, while high pH promotes full deprotonation and 8-coordinate lanthanide binding .
- Counterion effects : Chloride or acetate ions compete with carboxylate groups, altering complex stability constants by 1–2 orders of magnitude .
Q. How can slow complexation kinetics in cyclen-metal systems be addressed?
Kinetic bottlenecks arise from the transition from 4-coordinate (carboxylate-only) to 8-coordinate (NO) geometries. Strategies include:
- Pre-organization : Rigidifying the macrocycle with bulky substituents (e.g., tris-tBu-DOTA) reduces activation energy (ΔG ≈ 8.4 kcal/mol) .
- Microwave-assisted synthesis : Accelerates metal incorporation by enhancing ligand flexibility .
Q. How do structural variations in cyclen derivatives affect metal selectivity?
- Side-chain functionalization : Adding maleimide or acetamidobutyl groups (e.g., compound 17 ) enhances selectivity for Y, Ho, or Lu via steric and electronic effects .
- Hybrid ligands : Combining cyclen with phosphonic (DOTMP) or propionic (DOTPA) acids tunes metal affinity for therapeutic radionuclides (e.g., Y) .
Q. How to resolve contradictions in cyclen complex structural data?
Discrepancies in crystallographic data (e.g., torsional angles in 3 vs. 7 ) arise from intermolecular hydrogen bonding and packing effects. Multi-technique validation (NMR, X-ray, and DFT calculations) is critical for accurate conformational analysis .
Q. Methodological Guidance
- Handling and safety : Use nitrile gloves and P95 respirators to avoid dermal/ocular exposure. Store cyclen derivatives at 4°C under inert gas to prevent hydrolysis .
- Data interpretation : For NMR spectra, account for pH-induced line broadening by comparing with reference complexes (e.g., Lu-DOTA at pH 6.5) .
Properties
IUPAC Name |
1,4,7,10-tetrazacyclododecane | |
---|---|---|
Source | PubChem | |
URL | https://pubchem.ncbi.nlm.nih.gov | |
Description | Data deposited in or computed by PubChem | |
InChI |
InChI=1S/C8H20N4/c1-2-10-5-6-12-8-7-11-4-3-9-1/h9-12H,1-8H2 | |
Source | PubChem | |
URL | https://pubchem.ncbi.nlm.nih.gov | |
Description | Data deposited in or computed by PubChem | |
InChI Key |
QBPPRVHXOZRESW-UHFFFAOYSA-N | |
Source | PubChem | |
URL | https://pubchem.ncbi.nlm.nih.gov | |
Description | Data deposited in or computed by PubChem | |
Canonical SMILES |
C1CNCCNCCNCCN1 | |
Source | PubChem | |
URL | https://pubchem.ncbi.nlm.nih.gov | |
Description | Data deposited in or computed by PubChem | |
Molecular Formula |
C8H20N4 | |
Source | PubChem | |
URL | https://pubchem.ncbi.nlm.nih.gov | |
Description | Data deposited in or computed by PubChem | |
DSSTOX Substance ID |
DTXSID60183621 | |
Record name | Cyclen | |
Source | EPA DSSTox | |
URL | https://comptox.epa.gov/dashboard/DTXSID60183621 | |
Description | DSSTox provides a high quality public chemistry resource for supporting improved predictive toxicology. | |
Molecular Weight |
172.27 g/mol | |
Source | PubChem | |
URL | https://pubchem.ncbi.nlm.nih.gov | |
Description | Data deposited in or computed by PubChem | |
CAS No. |
294-90-6 | |
Record name | 1,4,7,10-Tetraazacyclododecane | |
Source | CAS Common Chemistry | |
URL | https://commonchemistry.cas.org/detail?cas_rn=294-90-6 | |
Description | CAS Common Chemistry is an open community resource for accessing chemical information. Nearly 500,000 chemical substances from CAS REGISTRY cover areas of community interest, including common and frequently regulated chemicals, and those relevant to high school and undergraduate chemistry classes. This chemical information, curated by our expert scientists, is provided in alignment with our mission as a division of the American Chemical Society. | |
Explanation | The data from CAS Common Chemistry is provided under a CC-BY-NC 4.0 license, unless otherwise stated. | |
Record name | Cyclen | |
Source | ChemIDplus | |
URL | https://pubchem.ncbi.nlm.nih.gov/substance/?source=chemidplus&sourceid=0000294906 | |
Description | ChemIDplus is a free, web search system that provides access to the structure and nomenclature authority files used for the identification of chemical substances cited in National Library of Medicine (NLM) databases, including the TOXNET system. | |
Record name | Cyclen | |
Source | EPA DSSTox | |
URL | https://comptox.epa.gov/dashboard/DTXSID60183621 | |
Description | DSSTox provides a high quality public chemistry resource for supporting improved predictive toxicology. | |
Record name | 1,4,7,10-tetraazacyclododecane | |
Source | European Chemicals Agency (ECHA) | |
URL | https://echa.europa.eu/substance-information/-/substanceinfo/100.102.391 | |
Description | The European Chemicals Agency (ECHA) is an agency of the European Union which is the driving force among regulatory authorities in implementing the EU's groundbreaking chemicals legislation for the benefit of human health and the environment as well as for innovation and competitiveness. | |
Explanation | Use of the information, documents and data from the ECHA website is subject to the terms and conditions of this Legal Notice, and subject to other binding limitations provided for under applicable law, the information, documents and data made available on the ECHA website may be reproduced, distributed and/or used, totally or in part, for non-commercial purposes provided that ECHA is acknowledged as the source: "Source: European Chemicals Agency, http://echa.europa.eu/". Such acknowledgement must be included in each copy of the material. ECHA permits and encourages organisations and individuals to create links to the ECHA website under the following cumulative conditions: Links can only be made to webpages that provide a link to the Legal Notice page. | |
Record name | 1,4,7,10-Tetraazacyclododecane | |
Source | European Chemicals Agency (ECHA) | |
URL | https://echa.europa.eu/substance-information/-/substanceinfo/100.118.281 | |
Description | The European Chemicals Agency (ECHA) is an agency of the European Union which is the driving force among regulatory authorities in implementing the EU's groundbreaking chemicals legislation for the benefit of human health and the environment as well as for innovation and competitiveness. | |
Explanation | Use of the information, documents and data from the ECHA website is subject to the terms and conditions of this Legal Notice, and subject to other binding limitations provided for under applicable law, the information, documents and data made available on the ECHA website may be reproduced, distributed and/or used, totally or in part, for non-commercial purposes provided that ECHA is acknowledged as the source: "Source: European Chemicals Agency, http://echa.europa.eu/". Such acknowledgement must be included in each copy of the material. ECHA permits and encourages organisations and individuals to create links to the ECHA website under the following cumulative conditions: Links can only be made to webpages that provide a link to the Legal Notice page. | |
Record name | CYCLEN | |
Source | FDA Global Substance Registration System (GSRS) | |
URL | https://gsrs.ncats.nih.gov/ginas/app/beta/substances/964584YO2O | |
Description | The FDA Global Substance Registration System (GSRS) enables the efficient and accurate exchange of information on what substances are in regulated products. Instead of relying on names, which vary across regulatory domains, countries, and regions, the GSRS knowledge base makes it possible for substances to be defined by standardized, scientific descriptions. | |
Explanation | Unless otherwise noted, the contents of the FDA website (www.fda.gov), both text and graphics, are not copyrighted. They are in the public domain and may be republished, reprinted and otherwise used freely by anyone without the need to obtain permission from FDA. Credit to the U.S. Food and Drug Administration as the source is appreciated but not required. | |
Synthesis routes and methods I
Procedure details
Synthesis routes and methods II
Procedure details
Synthesis routes and methods III
Procedure details
Retrosynthesis Analysis
AI-Powered Synthesis Planning: Our tool employs the Template_relevance Pistachio, Template_relevance Bkms_metabolic, Template_relevance Pistachio_ringbreaker, Template_relevance Reaxys, Template_relevance Reaxys_biocatalysis model, leveraging a vast database of chemical reactions to predict feasible synthetic routes.
One-Step Synthesis Focus: Specifically designed for one-step synthesis, it provides concise and direct routes for your target compounds, streamlining the synthesis process.
Accurate Predictions: Utilizing the extensive PISTACHIO, BKMS_METABOLIC, PISTACHIO_RINGBREAKER, REAXYS, REAXYS_BIOCATALYSIS database, our tool offers high-accuracy predictions, reflecting the latest in chemical research and data.
Strategy Settings
Precursor scoring | Relevance Heuristic |
---|---|
Min. plausibility | 0.01 |
Model | Template_relevance |
Template Set | Pistachio/Bkms_metabolic/Pistachio_ringbreaker/Reaxys/Reaxys_biocatalysis |
Top-N result to add to graph | 6 |
Feasible Synthetic Routes
Disclaimer and Information on In-Vitro Research Products
Please be aware that all articles and product information presented on BenchChem are intended solely for informational purposes. The products available for purchase on BenchChem are specifically designed for in-vitro studies, which are conducted outside of living organisms. In-vitro studies, derived from the Latin term "in glass," involve experiments performed in controlled laboratory settings using cells or tissues. It is important to note that these products are not categorized as medicines or drugs, and they have not received approval from the FDA for the prevention, treatment, or cure of any medical condition, ailment, or disease. We must emphasize that any form of bodily introduction of these products into humans or animals is strictly prohibited by law. It is essential to adhere to these guidelines to ensure compliance with legal and ethical standards in research and experimentation.