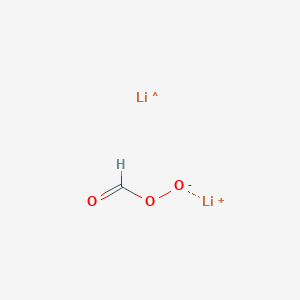
Lithium carbonate, 99.9% metal basis
- Click on QUICK INQUIRY to receive a quote from our team of experts.
- With the quality product at a COMPETITIVE price, you can focus more on your research.
Overview
Description
Lithium carbonate is an inorganic compound with the chemical formula Li₂CO₃. It is a white, odorless, crystalline powder that is widely used in various industries, particularly in the fields of medicine and energy storage. Lithium carbonate is highly valued for its role in the production of lithium-ion batteries, which power a wide array of electronic devices, from smartphones to electric vehicles .
Synthetic Routes and Reaction Conditions:
Carbonation Process: Lithium carbonate can be synthesized by reacting lithium hydroxide (LiOH) with carbon dioxide (CO₂) to form lithium carbonate and water.
Ion-Exchange Purification: High-purity lithium carbonate can be prepared using ion-exchange resins to remove impurities from lithium hydrocarbonate solutions.
Industrial Production Methods:
Extraction from Lithium Ores: Lithium carbonate is often produced from lithium-containing ores such as spodumene and lepidolite.
Salt Lake Brines: Another common method involves extracting lithium from salt lake brines.
Types of Reactions:
Thermal Decomposition: Lithium carbonate decomposes at high temperatures to form lithium oxide (Li₂O) and carbon dioxide (CO₂).
Acid-Base Reactions: Lithium carbonate reacts with strong acids like hydrochloric acid (HCl) to produce lithium chloride (LiCl), carbon dioxide (CO₂), and water (H₂O).
Common Reagents and Conditions:
Strong Acids: Hydrochloric acid (HCl) is commonly used to react with lithium carbonate in acid-base reactions.
High Temperatures: Thermal decomposition of lithium carbonate typically occurs at temperatures around 1000 K.
Major Products Formed:
Lithium Oxide (Li₂O): Formed during the thermal decomposition of lithium carbonate.
Lithium Chloride (LiCl): Produced when lithium carbonate reacts with hydrochloric acid.
Chemistry:
Battery Production: Lithium carbonate is a key precursor in the manufacture of lithium-ion batteries.
Biology and Medicine:
Mood Stabilizer: In the pharmaceutical industry, lithium carbonate is used as a mood stabilizer for the treatment of bipolar disorder.
Industry:
Mechanism of Action
The exact mechanism of action of lithium carbonate is not fully understood. it is believed to involve several molecular targets and pathways:
Inhibition of Enzymes: Lithium carbonate may inhibit enzymes such as glycogen synthase kinase 3 and inositol phosphatases.
Modulation of Neurotransmitters: It is thought to modulate neurotransmitter levels in the brain, particularly those involved in mood regulation.
Inositol Depletion Theory: This theory suggests that lithium carbonate acts as an uncompetitive inhibitor of inositol monophosphatase, leading to reduced levels of inositol and its derivatives.
Comparison with Similar Compounds
Lithium Citrate (Li₃C₆H₅O₇): Another lithium salt used in medicine, particularly for the treatment of bipolar disorder.
Lithium Orotate: Suggested to have better uptake properties and reduced toxicity compared to lithium carbonate.
Uniqueness of Lithium Carbonate:
Widespread Use in Batteries: Lithium carbonate is uniquely important in the production of lithium-ion batteries, which are essential for modern electronic devices.
Therapeutic Applications: It is one of the most commonly used lithium salts for the treatment of mood disorders.
Lithium carbonate’s versatility and effectiveness in various applications make it a critical compound in modern chemistry, medicine, and industry.
Properties
Molecular Formula |
CHLi2O3 |
---|---|
Molecular Weight |
75.0 g/mol |
InChI |
InChI=1S/CH2O3.2Li/c2-1-4-3;;/h1,3H;;/q;;+1/p-1 |
InChI Key |
MMSGMUNECPVYHR-UHFFFAOYSA-M |
Canonical SMILES |
[Li].[Li+].C(=O)O[O-] |
Origin of Product |
United States |
Disclaimer and Information on In-Vitro Research Products
Please be aware that all articles and product information presented on BenchChem are intended solely for informational purposes. The products available for purchase on BenchChem are specifically designed for in-vitro studies, which are conducted outside of living organisms. In-vitro studies, derived from the Latin term "in glass," involve experiments performed in controlled laboratory settings using cells or tissues. It is important to note that these products are not categorized as medicines or drugs, and they have not received approval from the FDA for the prevention, treatment, or cure of any medical condition, ailment, or disease. We must emphasize that any form of bodily introduction of these products into humans or animals is strictly prohibited by law. It is essential to adhere to these guidelines to ensure compliance with legal and ethical standards in research and experimentation.