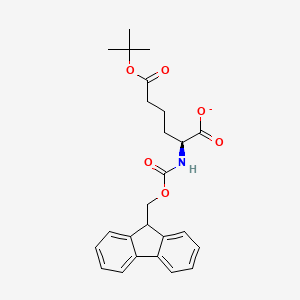
Fmoc-Aad(otBu)-OH
- Click on QUICK INQUIRY to receive a quote from our team of experts.
- With the quality product at a COMPETITIVE price, you can focus more on your research.
Overview
Description
Fmoc-Aad(otBu)-OH is a compound used in peptide synthesis. It is a derivative of amino acids, specifically designed for use in solid-phase peptide synthesis. The compound is protected by a fluorenylmethyloxycarbonyl (Fmoc) group at the amino terminus and a tert-butyl (otBu) group at the side chain. These protecting groups are essential for preventing unwanted reactions during peptide synthesis.
Preparation Methods
Synthetic Routes and Reaction Conditions
The synthesis of Fmoc-Aad(otBu)-OH typically involves the protection of the amino acid’s functional groups. The amino group is protected with the Fmoc group, while the side chain is protected with the otBu group. The synthesis can be carried out using standard peptide synthesis techniques, including the use of coupling reagents such as 2-(1H-benzotriazol-1-yl)-1,1,3,3-tetramethyluronium hexafluorophosphate (HBTU) and N,N-diisopropylethylamine (DIPEA) .
Industrial Production Methods
Industrial production of this compound involves large-scale synthesis using automated peptide synthesizers. These machines can perform the repetitive steps of deprotection and coupling efficiently, allowing for the production of large quantities of the compound. The use of high-purity reagents and solvents is crucial to ensure the quality of the final product .
Chemical Reactions Analysis
Types of Reactions
Fmoc-Aad(otBu)-OH undergoes several types of reactions, including:
Deprotection: Removal of the Fmoc group using piperidine.
Coupling: Formation of peptide bonds using coupling reagents.
Cleavage: Removal of the otBu group using trifluoroacetic acid (TFA).
Common Reagents and Conditions
Deprotection: Piperidine in dimethylformamide (DMF).
Coupling: HBTU and DIPEA in DMF.
Cleavage: TFA in the presence of scavengers like water and triisopropylsilane (TIS) .
Major Products Formed
The major products formed from these reactions are peptides with specific sequences, where this compound is incorporated at desired positions. The deprotected amino acid can further react to form longer peptide chains.
Scientific Research Applications
Supramolecular Chemistry
Self-Healing Gels
One of the most notable applications of Fmoc-Aad(otBu)-OH is in the development of self-healing gels. Research indicates that when combined with N-(3-dimethylaminopropyl)-N′-ethylcarbodiimide (EDC), this compound can form fibrillar networks that exhibit remarkable self-healing properties. The gel's recovery is attributed to the transient formation of cyclic anhydrides that can self-assemble into fibers due to π-stacking and hydrogen bonding interactions facilitated by the Fmoc group .
Mechanism of Action
The mechanism involves a chemical reaction cycle where this compound reacts with EDC to form a cyclic anhydride, which subsequently leads to the formation of a fibrillar network. The critical aggregation concentration (CAC) for this compound was determined to be approximately 0.5 mM, indicating its efficacy in forming stable assemblies in solution .
Drug Delivery Systems
This compound has potential applications in drug delivery due to its ability to form hydrogels that can encapsulate therapeutic agents. The hydrophobic nature of the Fmoc group enhances the loading capacity for hydrophobic drugs while allowing for controlled release profiles.
Case Study: Hydrogel Formation
In one study, hydrogels formed from Fmoc-modified peptides demonstrated excellent mechanical properties and stability, making them suitable for use as drug delivery vehicles. The gels can be tailored by adjusting the concentration of this compound and other co-assembling peptides, providing versatility in drug formulation .
Biocatalysis
The unique structural properties of this compound also lend themselves to applications in biocatalysis. By incorporating this compound into peptide-based catalysts, researchers have explored its ability to facilitate various chemical reactions under mild conditions.
Artificial Enzymes
Studies have shown that peptide assemblies derived from Fmoc-modified amino acids can mimic enzyme activity. For instance, hydrogels containing heme model compounds combined with Fmoc-modified peptides exhibited peroxidase-like activity, demonstrating their utility as artificial enzymes in catalysis .
Summary Table of Properties and Applications
Property/Application | Details |
---|---|
Chemical Structure | Fluorenylmethoxycarbonyl-protected alanine derivative with tert-butyl aspartic acid |
Self-Healing Gels | Forms fibrillar networks with EDC; exhibits recovery after damage |
Critical Aggregation Concentration (CAC) | Approximately 0.5 mM |
Drug Delivery Systems | Capable of encapsulating hydrophobic drugs; tunable release profiles |
Biocatalysis | Can mimic enzyme activity; used in artificial enzyme systems |
Mechanism of Action
The mechanism of action of Fmoc-Aad(otBu)-OH involves its incorporation into peptide chains during solid-phase synthesis. The Fmoc group protects the amino terminus, preventing unwanted reactions, while the otBu group protects the side chain. These protecting groups are removed at specific stages to allow for the formation of peptide bonds. The molecular targets and pathways involved depend on the specific peptide being synthesized and its intended application .
Comparison with Similar Compounds
Similar Compounds
Fmoc-Lys(otBu)-OH: Similar protecting groups but different side chain.
Fmoc-Orn(otBu)-OH: Similar protecting groups but different side chain.
Fmoc-His(Trt)-OH: Different side chain protection.
Uniqueness
Fmoc-Aad(otBu)-OH is unique due to its specific side chain protection, which allows for selective reactions during peptide synthesis. This makes it particularly useful for synthesizing peptides with specific functional groups .
Biological Activity
Fmoc-Aad(otBu)-OH, known as N-Fmoc-α-aminoadipic acid-δ-t-butyl ester, is a compound of interest in peptide synthesis and biological research. Its unique structure and properties make it a valuable building block in the design of bioactive peptides. This article reviews the biological activity of this compound, focusing on its synthesis, applications, and relevant research findings.
This compound has the molecular formula C₂₅H₂₉NO₆ and a molecular weight of 439.508 g/mol. The compound features a 9H-fluoren-9-ylmethoxy carbonyl (Fmoc) protecting group, which is commonly used in solid-phase peptide synthesis (SPPS) to protect the amino group during peptide assembly. The tert-butyl (t-Bu) ester provides stability and hydrophobicity, influencing the compound's solubility and interaction with biological systems.
Synthesis
The synthesis of this compound typically involves solid-phase peptide synthesis techniques, where protected amino acids are sequentially coupled to form peptides. The use of coupling agents like DIC (1,3-diisopropylcarbodiimide) is common in these reactions to activate carboxylic acids for amide bond formation. The process allows for the efficient assembly of peptides with desired sequences while maintaining high purity levels.
1. Self-Healing Properties
Recent studies have highlighted the self-healing capabilities of this compound when incorporated into supramolecular gels. Research demonstrated that solutions containing this compound could form fibrillar assemblies that exhibited self-healing characteristics upon mechanical disruption. In a study published in Chemical Science, confocal microscopy showed that these gels could recover their mechanical strength significantly after being damaged, indicating potential applications in drug delivery systems and tissue engineering .
2. Analgesic Activity
In vivo studies have explored the analgesic properties of peptides synthesized using this compound as a building block. A study examining various peptide analogs found that modifications in hydrophobicity and structure influenced analgesic activity in animal models. The results indicated that certain configurations led to enhanced pain relief compared to standard treatments .
Table 1: Summary of Biological Studies Involving this compound
Future Directions
The versatility of this compound in peptide synthesis opens avenues for further research into its biological activities. Future studies may focus on:
- Targeted Drug Delivery : Investigating how this compound-based peptides can be utilized in targeted therapies.
- Tissue Engineering : Exploring the self-healing properties in more complex biological environments.
- Structure-Activity Relationships : Systematically studying how variations in peptide structure influence biological outcomes.
Properties
Molecular Formula |
C25H28NO6- |
---|---|
Molecular Weight |
438.5 g/mol |
IUPAC Name |
(2S)-2-(9H-fluoren-9-ylmethoxycarbonylamino)-6-[(2-methylpropan-2-yl)oxy]-6-oxohexanoate |
InChI |
InChI=1S/C25H29NO6/c1-25(2,3)32-22(27)14-8-13-21(23(28)29)26-24(30)31-15-20-18-11-6-4-9-16(18)17-10-5-7-12-19(17)20/h4-7,9-12,20-21H,8,13-15H2,1-3H3,(H,26,30)(H,28,29)/p-1/t21-/m0/s1 |
InChI Key |
FFLAQPKWVSSKJC-NRFANRHFSA-M |
Isomeric SMILES |
CC(C)(C)OC(=O)CCC[C@@H](C(=O)[O-])NC(=O)OCC1C2=CC=CC=C2C3=CC=CC=C13 |
Canonical SMILES |
CC(C)(C)OC(=O)CCCC(C(=O)[O-])NC(=O)OCC1C2=CC=CC=C2C3=CC=CC=C13 |
Origin of Product |
United States |
Disclaimer and Information on In-Vitro Research Products
Please be aware that all articles and product information presented on BenchChem are intended solely for informational purposes. The products available for purchase on BenchChem are specifically designed for in-vitro studies, which are conducted outside of living organisms. In-vitro studies, derived from the Latin term "in glass," involve experiments performed in controlled laboratory settings using cells or tissues. It is important to note that these products are not categorized as medicines or drugs, and they have not received approval from the FDA for the prevention, treatment, or cure of any medical condition, ailment, or disease. We must emphasize that any form of bodily introduction of these products into humans or animals is strictly prohibited by law. It is essential to adhere to these guidelines to ensure compliance with legal and ethical standards in research and experimentation.