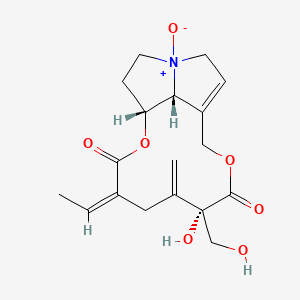
Riddelliine N-oxide
Overview
Description
Riddelliine N-oxide is a pyrrolizidine alkaloid, a class of naturally occurring compounds found in certain plants. These alkaloids are known for their toxic and carcinogenic properties. This compound is specifically found in plants of the genus Senecio, such as Senecio riddellii. It is a metabolite of riddelliine and shares similar toxicological properties .
Preparation Methods
Synthetic Routes and Reaction Conditions
The synthesis of Riddelliine N-oxide typically involves the oxidation of riddelliine. This can be achieved using various oxidizing agents under controlled conditions. One common method is the use of hydrogen peroxide in the presence of a catalyst to convert riddelliine to its N-oxide form .
Industrial Production Methods
the synthesis process in a laboratory setting can be scaled up for industrial purposes if needed, following similar oxidation procedures used in small-scale synthesis .
Chemical Reactions Analysis
Types of Reactions
Riddelliine N-oxide undergoes several types of chemical reactions, including:
Oxidation: Conversion of riddelliine to this compound.
Reduction: this compound can be reduced back to riddelliine under specific conditions.
Hydrolysis: In the liver, this compound can be hydrolyzed to form riddelliine.
Common Reagents and Conditions
Oxidizing Agents: Hydrogen peroxide, m-chloroperbenzoic acid.
Reducing Agents: Sodium borohydride, lithium aluminum hydride.
Hydrolysis Conditions: Enzymatic reactions in the liver involving cytochrome P450 enzymes.
Major Products Formed
From Oxidation: this compound.
From Reduction: Riddelliine.
From Hydrolysis: Riddelliine and other metabolites.
Scientific Research Applications
Toxicological Assessments
Genotoxicity and Carcinogenicity
Riddelliine N-oxide exhibits genotoxic properties comparable to its parent compound, riddelliine. Studies have indicated that while this compound is generally considered a detoxification product, it can still contribute to DNA adduct formation. For instance, in vitro studies with rat liver microsomes revealed a lower level of DNA adduct formation when exposed to this compound compared to riddelliine itself, suggesting that the N-oxide form may have reduced potency in inducing genetic damage .
Metabolic Pathways
Conversion Mechanisms
The metabolism of this compound involves its reduction back to riddelliine by intestinal microbiota. Research has shown that this conversion occurs rapidly and extensively in anaerobic conditions, indicating that the bioavailability and toxicity of this compound are significantly influenced by gut microbiota .
Physiologically based kinetic (PBK) modeling studies have quantified the relative potency (REP) of this compound compared to its parent compound. These models predict that the conversion rate of this compound decreases with higher doses due to saturation effects in the liver and intestines .
Case Studies and Research Findings
In Vivo Studies
A notable study utilized PBK modeling to assess the REP values of this compound in vivo. The findings indicated that oral administration of this compound resulted in a lower peak concentration (C_max) and a higher time to reach peak concentration (T_max) compared to riddelliine. Specifically, the area under the curve (AUC) for blood concentration showed a ratio of 0.67 when comparing both compounds, highlighting the reduced toxicity associated with the N-oxide form .
Human Health Implications
Research has also focused on understanding how interindividual differences in metabolism affect the toxicity of this compound. Variability in metabolic activation among individuals could lead to differing health outcomes upon exposure to foods containing this compound .
Analytical Techniques
Detection Methods
To study the presence and effects of this compound, various analytical methods have been employed:
Method | Description |
---|---|
High Performance Liquid Chromatography (HPLC) | Used for quantifying concentrations in biological samples. |
Gas Chromatography | Employed for analyzing volatile compounds related to riddelliine metabolites. |
Liquid Chromatography-Mass Spectrometry (LC-MS) | Allows for detailed analysis of metabolic pathways and product formation. |
These techniques are crucial for understanding both the pharmacokinetics and toxicodynamics of this compound.
Mechanism of Action
Riddelliine N-oxide exerts its effects primarily through its conversion to riddelliine in the liver. This conversion is mediated by cytochrome P450 enzymes. Riddelliine is then further metabolized to reactive intermediates, such as dehydropyrrolizidine (DHP), which can form DNA adducts. These adducts lead to mutations and ultimately carcinogenesis . The primary molecular targets are liver cells, where these DNA adducts are most commonly formed .
Comparison with Similar Compounds
Similar Compounds
Riddelliine: The parent compound of Riddelliine N-oxide, sharing similar toxicological properties.
Senecionine N-oxide: Another pyrrolizidine alkaloid N-oxide with similar toxic effects.
Retrorsine N-oxide: Similar in structure and toxicity to this compound
Uniqueness
This compound is unique due to its specific metabolic pathway and the formation of distinct DNA adducts that contribute to its carcinogenicity. Its presence in certain plants and its specific toxicological profile make it a compound of significant interest in toxicology and environmental studies .
Biological Activity
Riddelliine N-oxide, a metabolite derived from the pyrrolizidine alkaloid riddelliine, has garnered attention due to its biological activity, particularly its genotoxic and carcinogenic properties. This compound is primarily found in plants of the Senecio genus and has been implicated in various toxicological studies.
This compound is a phytochemical that exhibits structural similarities to its parent compound, riddelliine. Upon ingestion, this compound is metabolized in the liver and can be converted back to riddelliine through the action of intestinal microbiota . This metabolic pathway is crucial as it influences the compound's toxicity profile.
Genotoxicity and Carcinogenicity
Numerous studies have established that this compound possesses genotoxic activity comparable to that of riddelliine itself. It has been shown to induce DNA adduct formation, which is a critical step in the carcinogenic process. For instance, in vitro studies reported a lower level of DNA adducts when exposed to this compound compared to riddelliine, suggesting differential potency in their genotoxic effects .
Key Findings from Research Studies
-
In Vivo Studies :
- In Fischer 344 rats, this compound was administered at varying doses (0.01 to 1 mg/kg) over extended periods. The results indicated an increased incidence of hepatocellular tumors, particularly at higher doses .
- A study involving calves demonstrated that pure this compound caused significant morbidity at doses of 40.5 mg/kg/day, highlighting its toxic potential .
-
In Vitro Studies :
- Research has shown that this compound can be rapidly converted back to riddelliine by intestinal microbiota, which complicates the assessment of its toxicity .
- A comparative analysis indicated that the relative potency (REP) of this compound was approximately 67% of that of riddelliine when assessed through physiologically based kinetic (PBK) modeling .
Case Studies and Epidemiological Evidence
Epidemiological studies have linked exposure to pyrrolizidine alkaloids, including riddelliine and its N-oxide form, with various health issues:
- Liver Disease : Chronic exposure to these compounds has been associated with hepatic veno-occlusive disease in humans, particularly among those consuming herbal remedies containing Senecio species .
- Cancer Incidence : Increased incidences of liver tumors have been documented in animal models following long-term exposure to this compound, supporting its classification as a potential carcinogen .
Comparative Toxicity Data
Compound | Genotoxic Activity | Carcinogenic Potential | Relative Potency (REP) |
---|---|---|---|
Riddelliine | High | Confirmed | 1 |
This compound | Moderate | Likely | 0.67 |
Q & A
Basic Research Questions
Q. How should experimental designs account for dose discrepancies when comparing the toxicity of Riddelliine and Riddelliine N-oxide?
- Methodological Approach : Use equimolar dosing to ensure comparable pharmacokinetic profiles. Correct dose discrepancies by assuming a linear relationship between dose and DNA adduct formation, as seen in studies by Chou et al. (2003) and Wang et al. (2005). Include purity verification (e.g., 95–98% purity via HPLC) and anaerobic fecal cultures to model intestinal microbiota-mediated reduction of this compound .
- Key Considerations : Validate dose linearity using in vitro incubations and cross-reference with in vivo adduct quantification (e.g., LC-MS/MS or ³²P post-labeling) .
Q. What methodologies are recommended for quantifying DHP-derived DNA adducts in this compound toxicity studies?
- Methodological Approach : Prioritize LC-MS/MS for higher specificity and sensitivity, as demonstrated by Xia et al. (2013), which avoids overestimation biases associated with ³²P post-labeling. For cross-study comparisons, normalize adduct levels using dose-corrected REP values (Relative Potency) .
- Key Considerations : Ensure consistency in tissue sampling (e.g., liver microsomes) and account for sex-specific metabolic differences observed in rodent models .
Q. How does intestinal microbiota influence the bioactivation of this compound?
- Methodological Approach : Conduct anaerobic fecal incubations with fresh Wistar rat fecal samples to quantify reduction kinetics. Measure the rate of this compound conversion to Riddelliine under physiologically relevant conditions (pH, temperature) .
- Key Considerations : Compare reduction rates across species (e.g., human vs. rodent microbiota) to assess translational relevance .
Advanced Research Questions
Q. How can physiologically based kinetic (PBK) modeling predict in vivo REP values for this compound?
- Methodological Approach : Extend existing PBK models for Riddelliine (Chen et al., 2018) by incorporating compartments for this compound kinetics, including intestinal reduction, hepatic clearance, and glomerular filtration. Validate predictions against in vivo adduct data (e.g., Xia et al., 2013) and adjust for bioavailability assumptions (15% vs. 100%) .
- Key Parameters : Include Vmax and Km for hepatic enzymes, microbiota reduction rates, and species-specific blood flow rates .
Q. How can contradictions in REP values across studies (e.g., 0.36–0.64) be resolved?
- Methodological Approach : Perform sensitivity analysis to identify parameters causing variability (e.g., dose-dependent saturation of microbiota reduction or hepatic clearance). Use nonlinear regression to model AUC/dose ratios at varying doses, as saturation effects reduce REP values at higher doses .
- Key Insights : Discrepancies arise from methodological differences (e.g., ³²P vs. LC-MS/MS) and dose linearity assumptions. Standardize protocols using equimolar dosing and cross-validate with PBK predictions .
Q. What role does sensitivity analysis play in refining PBK models for this compound?
- Methodological Approach : Conduct linear sensitivity analysis on parameters affecting blood AUC predictions (e.g., hepatic clearance, intestinal reduction rates). Prioritize parameters with normalized sensitivity coefficients >0.1 (e.g., Vmax for liver enzymes, fecal reduction rates) .
- Key Outcomes : Identify critical pathways (e.g., hepatic metabolism contributes 60% to variability) and optimize experimental focus on high-sensitivity parameters .
Q. How do sex differences in rodent models impact the interpretation of this compound toxicity?
- Methodological Approach : Compare metabolic patterns in male vs. female rat liver microsomes. Track sex-specific differences in N-oxide formation and adduct profiles using LC-MS/MS. Correlate findings with hormonal or enzymatic variations (e.g., CYP450 isoforms) .
- Key Considerations : Extend PBK models to include sex-specific parameters for human risk assessment .
Q. What are the comparative toxicity profiles of this compound versus other pyrrolizidine alkaloid N-oxides?
- Methodological Approach : Normalize toxicity metrics (e.g., DNA adducts, hepatic clearance) across compounds using REP values. Reference comparative data (e.g., this compound has ~50% normalized toxicity vs. Riddelliine, whereas Senechionine N-oxide is ~10%) from structured toxicity hierarchies .
- Key Insights : Prioritize compounds with high REP values for combined exposure risk assessments .
Q. Methodological Standards & Validation
- Data Reporting : Follow NIH guidelines for preclinical studies, including detailed experimental conditions (e.g., microbiota sources, purity assays) and statistical validation of dose-response relationships .
- Model Transparency : Provide PBK model parameters in supplementary materials, including sensitivity coefficients and validation datasets, to enable reproducibility .
Properties
IUPAC Name |
(1R,4Z,7S,17R)-4-ethylidene-7-hydroxy-7-(hydroxymethyl)-6-methylidene-14-oxido-2,9-dioxa-14-azoniatricyclo[9.5.1.014,17]heptadec-11-ene-3,8-dione | |
---|---|---|
Source | PubChem | |
URL | https://pubchem.ncbi.nlm.nih.gov | |
Description | Data deposited in or computed by PubChem | |
InChI |
InChI=1S/C18H23NO7/c1-3-12-8-11(2)18(23,10-20)17(22)25-9-13-4-6-19(24)7-5-14(15(13)19)26-16(12)21/h3-4,14-15,20,23H,2,5-10H2,1H3/b12-3-/t14-,15-,18-,19?/m1/s1 | |
Source | PubChem | |
URL | https://pubchem.ncbi.nlm.nih.gov | |
Description | Data deposited in or computed by PubChem | |
InChI Key |
NPENPMDVCQGSSO-AHPXGCJSSA-N | |
Source | PubChem | |
URL | https://pubchem.ncbi.nlm.nih.gov | |
Description | Data deposited in or computed by PubChem | |
Canonical SMILES |
CC=C1CC(=C)C(C(=O)OCC2=CC[N+]3(C2C(CC3)OC1=O)[O-])(CO)O | |
Source | PubChem | |
URL | https://pubchem.ncbi.nlm.nih.gov | |
Description | Data deposited in or computed by PubChem | |
Isomeric SMILES |
C/C=C\1/CC(=C)[C@@](C(=O)OCC2=CC[N+]3([C@H]2[C@@H](CC3)OC1=O)[O-])(CO)O | |
Source | PubChem | |
URL | https://pubchem.ncbi.nlm.nih.gov | |
Description | Data deposited in or computed by PubChem | |
Molecular Formula |
C18H23NO7 | |
Source | PubChem | |
URL | https://pubchem.ncbi.nlm.nih.gov | |
Description | Data deposited in or computed by PubChem | |
DSSTOX Substance ID |
DTXSID501315962 | |
Record name | Riddelline N-oxide | |
Source | EPA DSSTox | |
URL | https://comptox.epa.gov/dashboard/DTXSID501315962 | |
Description | DSSTox provides a high quality public chemistry resource for supporting improved predictive toxicology. | |
Molecular Weight |
365.4 g/mol | |
Source | PubChem | |
URL | https://pubchem.ncbi.nlm.nih.gov | |
Description | Data deposited in or computed by PubChem | |
CAS No. |
75056-11-0 | |
Record name | Riddelline N-oxide | |
Source | CAS Common Chemistry | |
URL | https://commonchemistry.cas.org/detail?cas_rn=75056-11-0 | |
Description | CAS Common Chemistry is an open community resource for accessing chemical information. Nearly 500,000 chemical substances from CAS REGISTRY cover areas of community interest, including common and frequently regulated chemicals, and those relevant to high school and undergraduate chemistry classes. This chemical information, curated by our expert scientists, is provided in alignment with our mission as a division of the American Chemical Society. | |
Explanation | The data from CAS Common Chemistry is provided under a CC-BY-NC 4.0 license, unless otherwise stated. | |
Record name | Riddelliine N-oxide | |
Source | ChemIDplus | |
URL | https://pubchem.ncbi.nlm.nih.gov/substance/?source=chemidplus&sourceid=0075056110 | |
Description | ChemIDplus is a free, web search system that provides access to the structure and nomenclature authority files used for the identification of chemical substances cited in National Library of Medicine (NLM) databases, including the TOXNET system. | |
Record name | Riddelline N-oxide | |
Source | EPA DSSTox | |
URL | https://comptox.epa.gov/dashboard/DTXSID501315962 | |
Description | DSSTox provides a high quality public chemistry resource for supporting improved predictive toxicology. | |
Retrosynthesis Analysis
AI-Powered Synthesis Planning: Our tool employs the Template_relevance Pistachio, Template_relevance Bkms_metabolic, Template_relevance Pistachio_ringbreaker, Template_relevance Reaxys, Template_relevance Reaxys_biocatalysis model, leveraging a vast database of chemical reactions to predict feasible synthetic routes.
One-Step Synthesis Focus: Specifically designed for one-step synthesis, it provides concise and direct routes for your target compounds, streamlining the synthesis process.
Accurate Predictions: Utilizing the extensive PISTACHIO, BKMS_METABOLIC, PISTACHIO_RINGBREAKER, REAXYS, REAXYS_BIOCATALYSIS database, our tool offers high-accuracy predictions, reflecting the latest in chemical research and data.
Strategy Settings
Precursor scoring | Relevance Heuristic |
---|---|
Min. plausibility | 0.01 |
Model | Template_relevance |
Template Set | Pistachio/Bkms_metabolic/Pistachio_ringbreaker/Reaxys/Reaxys_biocatalysis |
Top-N result to add to graph | 6 |
Feasible Synthetic Routes
Disclaimer and Information on In-Vitro Research Products
Please be aware that all articles and product information presented on BenchChem are intended solely for informational purposes. The products available for purchase on BenchChem are specifically designed for in-vitro studies, which are conducted outside of living organisms. In-vitro studies, derived from the Latin term "in glass," involve experiments performed in controlled laboratory settings using cells or tissues. It is important to note that these products are not categorized as medicines or drugs, and they have not received approval from the FDA for the prevention, treatment, or cure of any medical condition, ailment, or disease. We must emphasize that any form of bodily introduction of these products into humans or animals is strictly prohibited by law. It is essential to adhere to these guidelines to ensure compliance with legal and ethical standards in research and experimentation.